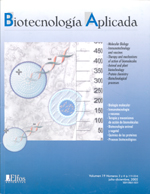
|
Biotecnologia Aplicada
Elfos Scientiae
ISSN: 0684-4551
Vol. 17, Num. 3, 2000, pp. 194
|
Biotecnología Aplicada 2000;194
Biotecnología Aplicada, Volume 17, July-September 2000, p. 194
Mass Spectrometry in Protein Studies from Genome
to Function
P Roepstorff
Department of Molecular Biology, Odense University, DK 5230 Odense
M, Denmark.
From a selection of papers from Biotecnología Habana`99 Congress.
November 28-December 3, 1999.
Code Number: BA00058
The triumphal progress of DNA sequencing
Advanced technology for determination of DNA sequences has become
widely available in the last decade and has been used for sequencing cDNA and
entire genomes from a variety of organisms ranging from viruses to human. As
a consequence, the amount of DNA sequence information entered in publicly accessible
databases has increased exponentially in the last decade. This growth has by
far exceeded the growth of sequences entered in databases based on protein sequencing.
In addition to the genomic sequencing, large-scale partial cDNA sequencing has
resulted in another set of data, the so-called expressed sequence tags containing
stretches of sequence from a large number of genes from a variety of organisms.
The genomic sequences, however, only provide information about the potencial
of the selected microorganisms and cell types but do not reflect the actual
situation at any given moment, i.e. which proteins are expressed and how they
are modified. cDNA sequences or the incomplete ESTs gives information on the
actually expressed proteins, but no information on processing and secondary
information. Therefore, studies of the proteins will never be obsolete.
Mass spectrometry has conquered protein analysis
Independently, but coinciding in time, mass spectrometric analysis
has undergone an equally dramatic development. From being an analytical tool
for analysis of small volatile molecules, new ionization methods, especially
electrospray ionization (ESI) [1] and matrix assisted laser desorption/ionization
(MALDI) [2], have increased the accessible mass range to include nearly all
proteins. Mass accuracy and sensitivity have been improved to routinely allow
molecular mass determination on the 100 ppm level or better of peptides
and proteins available in only mid to low femtomole amounts [3]. Mass spectrometry
(MS) has been proven ideal for analysis of peptide and protein mixtures and
partial or complete sequence information can be generated from the single components
in such mixtures by the so-called MS/MS techniques. In addition, MS is the ideal
technique for analysis of post translational modifications in proteins [4],
thus being the perfect complement to DNA sequencing.
Proteome analysis: the next step after the genome
Once a genome is sequenced, then the next natural step is analysis
of the proteome, which as defined by Wilkins et al. [5] represent:
"The total protein complement expressed by an organism, a cell or a tissue type".
Proteome analysis involves two essential steps. Firstly, separation and visualization
of the proteins and, secondly, identification of the proteins relative to the
genomic sequence, if known. 2-dimensional polyacrylamide gel electrophoresis
(2D-PAGE) is presently the only technique for separation of all or the majority
of the proteins from a given cell type. Identification of the proteins is now
routinely carried out by mass spectrometry after proteolytic digestion of the
proteins in the gel. This can be done either based on peptide maps produced
by MALDI MS or partial sequences produced by ESI MS (For a complete strategy
see: Shevchenko et al. [6], Jensen et al. [3]. Of these techniques
peptide mapping by MALDI MS is the simplest and most sensitive, whereas the
sequence based techniques are more specific. Partial sequences also often allow
identification in cases where only partial protein sequence information is available,
e.g., in EST databases [7]. Upon positive identification, the corresponding
cDNA can then be ordered and sequenced.
Characterization of secondary modifications is essential
Once a protein is identified, then the next obvious questions
are: Are the identified proteins post translationally modified and if so, how?
If the purified protein is available, then the strategy is to compare its molecular
mass determined by MS with that calculated based on the DNA sequence. If these
masses are different, then the modified sites and the types of modification
are identified based on mass spectrometric peptide mapping if relevant supplemented
with MS/MS of relevant peptide ions or degradation with appropriate enzymes,
e.g., glycosidases or phosphatases [8, 9]. If the proteins are only available
as spots or bands in electrophoretic gels, then it is often not possible to
determine the molecular mass of the intact protein, and characterization of
post translational modifications must rely on peptide mapping before and after
enzymatic treatments and when appropriate MS/MS. In such cases it is essential
to obtain complete or very high sequence coverage in the peptide maps [10, 11].
Studies of protein interaction and higher-order structures
The acceptance of mass spectrometry as a tool for studies of
protein interaction and protein higher-order structures is gradually increasing
[4]. Interaction screening based on "affinity fishing" followed by mass spectrometric
identification of the bound proteins shows great promise [12]. Studies of protein
conformation by deuterium exchange, of surface exposed residues by specific
labeling or limited proteolysis, and of the interaction interface by cross-linking,
although not competitive with techniques like X ray crystallography and NMR
spectrometry in terms of amount of detailed information, can yield valuable
information on much smaller protein quantities.
Conclusion
Mass spectrometry is now a viable tool on all levels in studies
of proteins from genome to function.
References
1. Fenn JB, Mann M, Meng CK, Wong SF, Whitehouse CM. Science
1989;246:6471.
2. Karas M, Hillenkamp F. Anal Chem 1988;60:22992301.
3. Jensen ON, Larsen MR, Roepstorff P. Proteins: Structure, Function
and Genetics 1998;999 Supl2:74-89.
4. Roepstorff P. Curr Opin Biotechnol 1997;8:613.
5. Wilkins MR, Pasquali C, Appel RD, Ou K, Golaz O, Sanchez J,
et al. Bio/Technology 1996;14:615.
6. Shevchenko A, Jensen ON, Podtelejnikov AV, Sagliocco F, Wilm
M, Vorm O, et al. Proc Nat Acad Sci (USA) 1996;93:144405.
7. Mann M. Trends Biol Sci 1996;21:4945.
8. Burlingame AL. Curr Opin Biotechnol 1996;7:410.
9. Bean MF, Annan RS, Hemling ME, Mentzer M, Huddleston MJ, Carr
SA. In Techniques in Protein Chemistry VI. Crabbe, J., (ed.) Academic Press,
San Diego 1995;pp:10716.
10. Moertz E, Saraneva T, Haebel S, Julkunen l, Roepstorff P.
Electrophoresis 1996;17:1493502.
11. Wilm M, Neubauer G, Mann M. Anal Chem 1996a;68:52733.
12. Nordhoff E, Krogdam AM, Joergensen HF, Kallipolitis BH, Clark BFC, Roepstorff
P. Nature Biotechnology 1999;17:8848.
Copyright Elfos Scientiae 2000
|