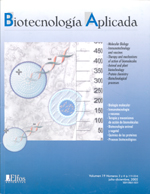
|
Biotecnologia Aplicada
Elfos Scientiae
ISSN: 0684-4551
Vol. 16, Num. 1, 1999
|
ba99002
Biotecnologia Aplicada 1999; Vol. 16 No. 1, pp.11 - 14
Plant Regeneration From Leaf
And Stem
Explants From Two Sweet Potato
(Ipomoea batatas L. Lam.) Cultivars
Rolando García González, Danalay
Somontes Sánchez, Jesús Mena Campos,
Eulogio Pimentel Vázquez, Zurima Zaldúa Guerra, Alina
López Quesada,
Rolando Morán Valdivia, Melba García
González
División de Plantas. Centro de Ingeniería
Genética y Biotecnología. Camagüey. AP 387, CP
70100. Camagüey, Cuba. Tel: (53-322) 61295; Fax: (53-322)
61587; E-mail: invest@cigbcam.cigb.edu.cu
Code Number: BA99002
ABSTRACT
An efficient protocol for organogenic regeneration of two
sweet potato (Ipomoea batatas L.) elite cultivars, was
developed. Several growth regulator combinations and explant
sources were tested, so an efficient protocol for plant
regeneration was established. Out of 151 plant growth regulator
combinations, three were able to induce the highest percentage of
shoot and root formation for both cultivars. The first medium was
supplemented with 1.0 mg/L naphthalene acetic acid (NAA) and
0.1 mg/L benzylaminopurine; the second, with 1.0 mg/L
paclobutrazol and 0.1 mg/L NAA; and the third, with
0.5 mg/L indol-3-acetic acid.
Keywords: growth regulators, Ipomoea batatas,
plant regeneration, sweet potato, tissue culture
RESUMEN
En este trabajo reportamos la obtención de una
metodología para la regeneración de dos variedades
comerciales de boniato (Ipomoea batatas L.). Para realizar
nuestro estudio, ensayamos diferentes combinaciones de reguladores
de crecimiento, así como distintas fuentes y tipos de
explantes. Sólo tres combinaciones de las 151 variantes
ensayadas fueron capaces de inducir la formación de brotes
aéreos y raíces con una alta frecuencia. El primer
medio corresponde a la combinación de ácido
naftalenacético (ANA) a una concentración de
1,0 mg/L y benzilaminopurina a 0,1 mg/L en medio MS; la
segunda combinación correspondió a la adición
de 1,0 mg/L de paclobutrazol y 0,1 mg/L de ANA al medio
MS; y la tercera variante, con resultados satisfactorios, fue el
medio MS suplementado con 0,5 mg/L de ácido
3-indolacético.
Palabras claves: boniato, cultivo de tejidos, Ipomoea
batatas, regeneración de plantas,reguladores del
crecimiento
Introduction
Sweet potato (Ipomoea batatas L.) ranks seventh among
all food crops worldwide, with an annual production of 115 million
metric tons. Of the root and tuber crops, the sweet potato ranks
third in acreage (7.9 million ha) behind the potato and cassava
[1]. Sweet potato is grown in more than 100 countries and among the
world´s root and tuber crops, it ranks second in importance [2]. It
is consumed as a fresh vegetable (roots, petioles, leaves and
stems), staple food, snack food and it is also used for industrial
starch extraction and fermentation [3-6]. Sweet potato is
industrially dehydrated [7] and used as an important component of
bread flour [8].
Although the sweet potato is one of the most important plants in
the world, biotechnological work on it has lagged behind. In recent
years a few groups have reported their experience in the molecular
biology manipulation of sweet potato to increase nutritional
quality [9] and pest resistance [10]. Others have been working on
its cryopreservation [11], protoplast isolation and regeneration
[12, 13], and its regeneration and transformation from roots,
petioles, stems and leaves [14-17].
Sweet potato is more tolerant and needs less nutrients from the
soil than most of the crops, being therefore, one of the most
cultivated crops in developing countries. However, the increase in
production is limited due to the severe damages caused by pests and
diseases. In many regions where sweet potatoes are produced in
low-input agricultural systems, insect related losses may often
reach 60-100% [18].
The sweet potato weevil (Cylas spp.) is the major
biological antagonist of sweet potatoes worldwide. Controlling is
quite difficult and the improvement programs developed have only
been partially successful. The improved cultivars do not show a
stable performance in different ecological conditions; most of them
depend on the soil structure or the physiological and botanical
features of the cultivar rather than on the genetic production of
chemical defenses against the pest [19, 20]. Both of the above
mentioned reasons and the lack of any strong conventional genetic
improvement program to obtain pest resistance or to increase its
nutritional quality, make the sweet potato an important target to
be modified by biotechnological tools.
It is recalcitrant to regeneration and transformation because
each cultivars shows different responses to in vitro
treatments. Several protocols reporting sweet potato regeneration
[10, 14-16, 21] were tested, in order to establish an efficient
transformation methodology, but they failed to work for our
cultivars under our conditions. Every cultivar vary widely in their
response to tissue culture and plant regeneration [14, 15]. Hence,
the genotype is one of the most important factors affecting the
evolution of the in vitro culture, regeneration and
transformation responses [14, 15, 21, 22].
The latest and most successful protocol obtained for the
regeneration and Agrobacterium tumefaciens mediated
transformation of sweet potato was reported by Newell, et
al. from the University of Bath in 1995 [10].
The target explants used for the Newell group were tuberous
roots grown in greenhouses where many conditions were tested to
induce regeneration from the meristematic bud-like centers (MBLC),
potentially existing in the tuberous root tissue. Transgenic shoots
were obtained following this methodology at 16 weeks of culture
after the transformation step. The shoots showed to be transformed
when tested. Newell´s paper was the first report of the
transformation of commercial sweet potato cultivars with an
economically interesting gene; the previous papers were only on the
introdutcion of genes into the sweet potato genome. However,
Newell´s methodology is too difficult to reproduce because the
target explant used is very hard to clean and to adapt to the in
vitro culture conditions. Additionally disadvantageous is that
the protocol reported by Newell took a long time to regenerate
transgenic shoots. The regeneration and transformation frequencies
shown in the article were about 5%, but the number of explants
manipulated during the experiments were very high.
The aim of this paper is to establish an efficient methodology
for sweet potato regeneration to make the following transformation
steps possible. The target explants used were leaves and stems. The
first transgenic shoots via organogenesis were obtained three to
four weeks after cultivation on the regeneration medium. Two very
important cultivars were studied in our experiments: Jewel and
CEMSA-78354. Our study was separated into two steps. As a first
step different growth regulator combinations and three sources of
explants were tested in order to establish an efficient medium for
shoot induction. The second step included certain factors that
could affect the transformation such as: induction of vir
genes, time of cocultivation, temperature, etc, which are currently
tested.
Materials and Methods
Vegetable material
Two genotypes were used during all the experiments: Jewel, a
very important commercial genotype cultivated in North and South
America, and CEMSA-78354, the most commonly used in Cuba. Both were
supplied by the collection of the Instituto Nacional de
Investigaciones en Viandas Tropicales (INIVIT) in Santo Domingo,
Cuba. The in vitro plants are kept in an MS [23] solid
medium supplemented with Sucrose 3%, IAA 0.5 mg/L, agar-agar 6.0
g/L and pH 5.6-5.7 adjusted before autoclaving.
Regeneration conditions
Leaves and stems were taken from young plants grown in glass
culture tubes containing 7 mL of the MS medium supplemented
with sucrose 3%, nicotinic acid 0.5 mg/L, thyamine-HCl
0.1 mg/L, piridoxine-HCl 0.5 mg/L, glycine 2 mg/L,
myo-Inositol 100 mg/L, indol-3-acetic acid 0.5 mg/L
(aidded prior autoclaving). The pH was adjusted to 5.6-5.7 before
autoclaving (20 min; 121 ºC; 138 kPa). The in
vitro plants were kept for six weeks at 25 ºC in a 12/12
photoperiod, 66% relative humidity.
All experiments were carried out in 10 cm petri dishes
containing 25 mL of each regeneration medium. All the
experiments were randomly set up in six replicates for each growth
regulator combination. Each replicates consisted of 6 leaves and 10
stems. The explants were incubated at 25 ºC and in a 12 h
photoperiod and all were subcultured in a fresh medium every 15
days. The final evaluation of the experiment was performed after
six weeks. All treatments were assessed according to their
influence on the tissues' responses to regeneration and
differentiation, following the criteria described below.
The basal medium for all studies was the MS basal salt mixtures
and vitamins supplemented with 3% sucrose and solidified with agar
noble Difco (Difco Laboratories Inc.). The pH was adjusted to
5.6-5.7 before autoclaving (20 min; 121 ºC;
138 kPa). All growth regulators were added prior to
autoclaving, except zeatin riboside, which was added filter-sterile
to the cooling medium.
We used the whole leaf trying to keep a 2-5 mm piece of
petiole; the leaves were placed and expanded on the media. Stems
were removed very carefully, trying not to extract any vegetative
bud; there was no regular size for the stem pieces, since we used
the internodal section without considering its size.
Evaluation criteria
To select the best regeneration conditions we studied the
influence of each treatment on: calli formation, root regeneration
and shoot emission. We calculated the regeneration efficiency of
direct shoots as follows:
RE = (RS / TNE) x 100
where:
RE: percentage of regeneration efficiency of direct
shoots
RS: total number of regenerated shoots
TNE: total number of tested explants
The term regeneration frequency was introduced to determine the
functional shoot or root emission per explant. It was calculated as
follows:
RF = NRP / TNE
where:
RF: regeneration frequency per explant
NRP: number of rooted functional shoots
TNE: total number of tested explants
Regeneration studies on solid medium
Sweet potatoes are reported to have a genotype dependent
response both to organogenesis and somatic embryogenesis. Therefore
a wide number of growth regulators have been tested for their
influence on several sweet potato genotypes and they have shown
different patterns in calli formation and shoot and root emission
[10, 14-16]. Several growth regulator combinations reported as
having different effects on sweet potato regeneration were tested.
Combining auxins and cytokinins or using them alone in the medium
were the basic treatments tested to achieve high root and shoot
emission.
Growth regulator combinations
a) First experiment. Thirty five
combinations of two growth regulators at different concentrations
were tested: [indol-3-acetic acid (IAA) (0.0 mg/L,
0.25 mg/L, 0.5 mg/L, 0.75 mg/L, 1.0 mg/L,
1.25 mg/L, 2.0 mg/L) and zeatin riboside (0.11 mg/L,
0.22 mg/L, 0.5 mg/L, 0.75 mg/L, 1.0 mg/L)]. The
explants were cultured on these combinations for six weeks at
25 ºC and a 12/12 h photoperiod. All the explants were
subcultured in a fresh medium every 15 days. Partial evaluations
every 10 days and a final evaluation at the end of the experiments,
were performed. The general culture conditions were the same for
the next experiments.
b) Second experiment. Another cytokinin growth
regulator was tested combined with IAA, instead of zeatin. The IAA
levels and the conditions for this study were the same as for the
first experiment. Kinetin riboside was used as cytokinin at
different concentrations (0.1 mg/L, 0.5 mg/L,
1.0 mg/L, 2.0 mg/L). 28 combinations were
used.
c) Third experiment. 6-benzylaminopurine (BAP)
at 0.25 mg/L, 0.5 mg/L, 1.0 mg/L, 2.0 mg/L and
3.0 mg/L was used combined with AIA at similar levels to the
preceding experiments. The experimental conditions were stable for
this and the next two experiments. 35 combinations were
tested.
d) Fourth experiment. Twenty variants were
tested in this experiment. Auxin was changed for this study and BAP
was kept at the same levels as the above cytokinin. Naphthalene
acetic acid (NAA) was added at: 0.1 mg/L, 0.5 mg/L, 1.0 mg/L and
2.0 mg/L.
e) Fifth experiment. NAA was combined with
kinetin at 0.1 mg/L, 0.5 mg/L, 1.0 mg/L and
2.0 mg/L. Six variants were studied from the combinations of
these regulators at the concentrations described.
f) Sixth experiment. A new growth regulator,
unreported for sweet potato tissue culture, was used in this study.
Paclobutrazol (PPP) is a chemical that blocks the giberelic acid
pathway, thus, dominance exclusion is expected when it is supplied
to the plant. For sweet potato this is a very common phenomenon. In
previous studies we demonstrated that the first shoots and roots
regenerated from leaf explants restrained other shoot and root
formations on the same explants. When the first regenerated shoots
were removed from the explants, other shoots or roots were formed
from the original explants. In this experiment NAA at
0.0 mg/L, 0.1 mg/L, 0.5 mg/L, 1.0 mg/L,
2.0 mg/L and PPP at 0.0 mg/L, 1.0 mg/L,
2.0 mg/L, 3.0 mg/L, were combined. PPP was added to the
medium before pH was adjusted to 5.6-5.7; then it was coautoclaved
at 120 ºC and 138 kPa for 20 min. L-cysteine was added to
40 mg/L and ascorbic acid to 80 mg/L was added after
sterilization, to avoid plant tissue oxidation. The explants were
kept on these combinations for six weeks and were then evaluated.
PPP and NAA combinations resulted in 20 variants.
Results and Discussion
It was difficult to analyze all the data collected after
such a large combination of variants. The number of shoots and
roots regenerated, and the regeneration frequency (shoots or root
regenerated on the total number of explants), were considered for
the selection of a competent condition for sweet potato
regeneration. As in all the regeneration and transformation
protocols it is very important to have high plant conversion in
order to increase the likelihood of transformed plants.
Leaves had a higher regeneration frequency compared to stem.
Therefore, we consider that leaves are suitable target explants in
the transformation studies based on this organogenesis mediated
protocol. The most potential area for shoot and root formation was
just above the cut piece of petiole on the leaf where a small
callus was formed before the emission of roots or shoots, or
both.
The stem explants were able to form non-embryogenic calli on the
cut side of the explants but early organogenic shoots appear before
these calli and they were always regenerated from the meristematic
area placed in the nodal section. The CEMSA-78354 stems were harder
to manipulate than the Jewel since in the former, the internodal
segments are very short and the vegetative buds may have not been
completely removed. As a result of this handicapped shoot emission,
more than 95% were obtained for the CEMSA-78354 and 70% for the
Jewel emissions, on several media from both cultivars (MS + IAA
0.5 mg/L + sucrose 3%; MS + sucrose 3% + BAP 0.1 mg/L +
NAA 1.0 mg/L; MS + sucrose 3% + Kin 0.1 mg/L + IAA
0.5 mg/L). However, it is believed that this was due to some
remaining meristematic and differentiated tissues from the buds
that continued developing into normal plants under the growth
regulator effects. Although not yet demonstrated, the classical
organogenesis and bud development was observed only a week after
culturing the explants under the above mentioned conditions.
Remarkably, the influence of auxin to induce direct
organogenesis was observed to be greater than that one of the
cytokinins. More stimulation for non embryogenic calli formation
were usually found when the explants were treated with cytokinins
(even at low concentrations) compared to auxins. In some cases
shoots were achieved by giving moderated auxin treatments (IAA
0.5 mg/L; NAA 0.1 mg/L). There is no previous reference
of using auxin based stimulation alone to induce plant regeneration
in sweet potato, especially for these cultivars.
The CEMSA-78354 cultivar was more difficult to regenerate than
the Jewel. This cultivar was induced to form calli under low levels
of any kind of growth regulator. The calli were compact and hard to
manipulate with blades, they were green throughout the area that
was in full contact with the medium, but the cells on the top were
always white. Root emission and sporadic shoot formation were
obtained as well, but at a very low frequency on a growth regulator
free MS medium. This must be due to the endogenous growth
regulators for this cultivar. Secondary roots were formed profusely
in 127 treatments assayed and it is thought to be a very important
fact blocking shoot formation. When the first roots or shoots
emitted were separated from the original explants, new roots or
shoots were formed again.
Shoot induction both from the original explant and the
regenerated root were achieved on several combinations of growth
regulators during the regeneration study. However, none was above
20% of regeneration, except for three of the growth regulator
combinations studied on which the following observations were
based. The most significant results were obtained for the following
three combinations (Tables 1-3).
Table 1. Regeneration on a BN-3 medium [MS
+ sucrose (3%) + BAP (0.1 mg/L) + NAA (1.0 mg/L)]. Note that the
Jewel cultivar behaved better than the CEMSA-78354.
Cultivar |
Direct shoots (%) |
Rooting |
Indirect shoots |
Regeneration frequency |
Jewel |
68.90 |
+ + + |
100.00 |
0.70 |
CEMSA-78354 |
13.75 |
+ + + |
3.00 |
0.14 |
+: moderated; + +: good; + + +: high
Table 2. Regeneration on a PN-2 medium [MS
+ sucrose (3%) + PPP (1.0 mg/L) + NAA (1.0 mg/L)].
Cultivar |
Direct shoots (%) |
Rooting |
Indirect shoots |
Regeneration frequency |
Jewel |
85.00 |
+ + + |
100.00 |
0.85 |
CEMSA-78354 |
27.50 |
+ + + |
12.00 |
0.27 |
+: moderated; + +: good; + + +: high
Table 3. Regeneration on a MPM medium [MS
+ sucrose (3%) + IAA (0.5 mg/L). Note that the frequency of shoot
per explants for Jewel was above 2.
Cultivar |
Direct
shoots (%) |
Rooting |
Indirect shoots |
Regeneration frequency |
Jewel |
200.00 |
+ + + |
100.00 |
3.0 |
CEMSA-78354 |
22.50 |
+ + + |
12.50 |
0.3 |
+: moderated; + +: good; + + +: high
The direct shoots are formed both by organogenesis or
embryogenesis, from the original explants. Indirect shoots are
regenerated from de novo roots formed from the original
explants. More than one shoot were usually obtained from the
regenerated roots for the Jewel cultivar. Of greater importance was
the indirect shoot formation in CEMSA-78354: 92.8% of the shoots
obtained were induced from the regenerated roots.
Certain authors have reported the formation of MBLC [10] in the
tuberous roots, but none of the studies reported mentioned this
phenomenon for the regenerated roots. These indirect shoots started
as organized dark red cells on the surface of the roots and were
rapidly differentiated into plants by organogenesis. The shoots
formed were capable of rooting in the MPM propagation medium.
The influence of PPP on sweet potato regeneration was assessed.
As the elimination of dominance is expected when PPP is in the
medium, the frequency of shoots per explant can be increased. This
has been proven for both cultivars. The number of roots emitted per
explant was over 5 when the explants were treated with a
concentration above 0.5 mg/L of PPP.
On the other hand, the number of roots forming indirect shoots
was higher for these treatments. However, severe tissue oxidation
and non-functional shoots were usually observed when the PPP
concentration was over 1 mg/L, specially for CEMSA-8354.
As already stated, the CEMSA-78354 cultivar was more difficult
to regenerate than Jewel. All the explants tested were easily
induced to form calli under low levels of any kind of the growth
regulators tested. Root emission was achieved even on growth
regulator-free MS medium. Secondary roots were formed profusely and
they are thought to block shoot formation in many of the treatments
assayed; in fact, the emission of new roots and shoots was
stimulated when the first roots and shoots were cut out from the
explant. Besides, a high number of explants developed more than one
shoot, but only one was able to grow unless they were detached from
each other. When the most developed shoots were taken from the
original explant, the shortest ones started to develop into normal
plants. Although the dominance effect was maintained, it was less
important than that induced for the first shoot.
Again, the differences between two sweet potato cultivars was
shown, now in all of our experiments, which supports the previous
studies that were limited to several conditions for one cultivar
[24] and are limited for other cultivars.
Finally, it is underlined that no embryogenic response was
observed in any of the studies. All the shoots and roots were
achieved by direct or indirect organogenesis. Embryogenesis for
sweet potato has always been obtained giving long 2,4-D treatments
[10, 12, 23], but it has not worked for our cultivars. Calli
started growing very quickly and they were formed by globular
structures during the second week for the earliest treatment and at
the sixth for the last. However, these structures became
undifferentiated two weeks after their formation and they
regenerated into a root or a shoot in all the experiments without
completing the last embryogenic steps.
The regenerated shoots were always planted in the MPM medium for
rooting and they developed whole plants for both cultivars. These
plants did not differ in their phenotype with the wild in
vitro cultured plants adapted to greenhouse conditions.
R eferences
FAO. FAO Production Year Book. Food and Agriculture
Organization. Rome: 1984.
Horton DE. Potatoes: production, marketing and programs for
developing countries. Colorado: West Press, Boulder; 1987.
Bouwkamp JC. Introduction. In: Bouwkamp JC, editor. Sweet
potato products: a natural resource for the tropics. Boca Raton,
Florida: CRC Press; 1985. p.3-7.
Kays SJ. Formulated sweet potato products. In: Bouwkamp JC,
editor. Sweet potato products: a natural resource for the tropics.
Boca Raton, Florida: CRC Press; 1985. p.205-18.
Lin SSM, Peet CC, Chen DM, Lo HF. Sweet potato production and
utilization in Asia and the Pacific. In: Bouwkamp JC,
editor. Sweet potato products: a natural resource for the tropics.
Boca Raton, Florida: CRC Press; 1985. p.139-48.
Sakamoto S, Bouwkamp JC. Industrial products from sweet potato.
In: Bouwkamp JC, editor. Sweet potato products: a natural resource
for the tropics. Boca Raton, Florida: CRC Press; 1985.
p.219-34).
Ensminger AH, Ensminger ME, Konlande JE, Robson JRK. Sweet
potato, the concise encyclopedia of foods & nutrition.
CRC Press; 1995. p.1001-2).
López Zada M, Vázquez E, Becalli R, López
F. Raíces y tubérculos. La Habana: Pueblo y
Educación; 1995.
López A, Zaldúa Z, Pimentel E, García M,
García R, Mena J, et al. Modification of sporamin
gene from sweet potato with a synthetic DNA fragment. Nucleotide
sequence and expression in E. coli. Biotecnología
Aplicada 1996;13:265-70.
Newel CA, Lowe JM, Merryweather A, Rooke LM, Hamilton WDO.
Transformation of sweet potato (Ipomoea batatas (L.) Lam.)
with Agrobacterium tumefaciens and regeneration of plants
expressing cowpea trypsin inhibitor and snowdrop lectin. Plant
Science 1995;107:215-27.
Blakesley D, Al Mazrooei S, Bhatti MH, Henshaw GG.
Cryopreservation of non-encapsulated embryogenic tissue of sweet
potato (Ipomoea batatas). Plant Cell Reports
1996;15:873-6.
Sihachakr D, Ducreux G. Plant regeneration from protoplast
culture of sweet potato (Ipomoea batatas Lam.). Plant Cell
Rep 1987;6:326-8.
Otani M, Shimada T, Niizeiki H. Mesophyll protoplast culture of
sweet potato (Ipomoea batatas L.). Plant Science
1987;53:157-60.
Gosukonda RM, Prakash CS, Porobodessai A, Blay E, Peterson CM.
Thidiazuron-induced adventitious shoot regeneration of sweet potato
(Ipomoea batatas), in vitro Cell Dev Biol
1995;31:65-71.
Gosukonda RM, Prakash CS, Porobodessai A. Shoot regeneration
in vitro from diverse genotypes of sweet potato and multiple
shoot production per explant. HortScience 1995;30 Suppl
5:1074-77.
Carswell GK, Locy RD. Root and shoot initiation by leaf, stem,
and storage root explants of sweet potato, Plant Cell Tissue and
Organ Culture 1984;3: 229-36.
Otani M, Mii M, Handa T, Kamada A, Shimada T. Transformation of
sweet potato (Ipomoea batatas (L) Lam.) plants by
Agrobacterium rhizogenes. Plant Science 1993;94:151-9.
Chalfant RB, Jansson RK, Seal DR. Ecology and Management of
sweet potato insects. Annu Rev Entomol 1990;35: 157-80.
Sutherland JA. A review of the biology and the control of the
sweet potato weevil Cylas formicarius (Fab). Trop Pest
Manage 1986;32:304-15.
Villareal RL, Grigs TDL, editors. Sweet potato in the
tropics-progress and problems. Sweet potato, Proc. 1st Int. Symp.,
Asian Veg Res Dev Cent; Tainan, Taiwan, Republic of China;
1982.
Prakash CS, Varadarajan U. Genetic transformation of sweet
potato by particle bombardment. Plant Cell Reports
1992;11:53-7.
Jarret RL, Gawel N. Chemical and environmental growth of sweet
potato (Ipomoea batatas (L.) Lam.) in vitro. Plant
Cell, Tissue and Organ Culture 1991;25:153-9.
Murashige T, Skoog F. A revised medium for rapid growth and
bioassays with tobacco tissues cultures. Physiol Plant
1962;15:473-97.
Schulheis RJ, Cantliffe JD, Chee RP. Optimizing sweet potato
(Ipomoea batatas L. Lam.) root and plant formation by
selection of proper embryo developmental stage and size, and gel
type for fluidized sowing, Plant Cell Reports
1990;9:356-7.
Copyright 1999 Elfos Scientiae
|