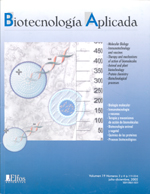
|
Biotecnologia Aplicada
Elfos Scientiae
ISSN: 0684-4551
Vol. 16, Num. 2, 1999
|
BA99021
Biotecnología Aplicada 1999;16:127-130
Genetically Modified Fish in Aquaculture: Technical,
Environmental and Management Considerations
Peter Aleström,1@ José de la Fuente2
1Department of Biochemistry, Physiology and Nutrition, Norwegian
College of Veterinary Medicine.
PO Box 8146 DEP, N-0033 Oslo, Norway.
2División de Genética de Células de
Mamíferos, Centro de Ingeniería Genética y
Biotecnología.
AP 6162, CP 10600, Ciudad de La Habana, Cuba.
Telef: (53-7) 21 8164; Fax: (53-7) 21 8070; E-mail:
jose.delafuente@cigb.edu.cu
Code Number:BA99021
ABSTRACT
Genetically modified (GM) fish offer new possibilities for the
improvement of production in aquaculture. It allows the
introduction of novel traits or the improvement of old ones, in
such a way that is out of reach for classical selection breeding.
Examples of genes with commercial potential are among those which
control growth, disease resistance, freeze tolerance, sexual
maturation, food quality and food preservation parameters.
Consumption of GM fish does not represent a health risk in
principle. The safety of GM food is dependent on the character of
the transgene, the transgene product and the new phenotype. Ethics
and animal protection concerns demand the development of healthy
fish only. Environmental safety calls for efficient biological
containment in order to minimize possible effects caused by
released farm animals. Improvements of disease control will support
both production economy and the environment, in case of escapes.
Since aquaculture includes both marine and fresh water species, it
can be developed as new food production strategies in most
countries all over the world. To avoid large-scale technology
transfer failures, it is important to adapt to the regional and
local needs. This calls for international research collaboration
aiming at regional and local competence development sufficient for
the technology implementation.
Keywords: aquaculture, fish, safety, transgenic
RESUMEN
Los peces modificados genéticamente (MG)
brindan nuevas posibilidades para el desarrollo de la
producción acuícola. Esta tecnología permite
la introducción y modificación de caracteres
genéticos de una forma mucho más rápida y
eficiente que los procesos clásicos de selección.
Entre los genes con posibilidades comerciales, se encuentran
aquellos que controlan el crecimiento, la resistencia a
enfermedades, la tolerancia al frío, la maduración
sexual, la calidad de la carne, etc. El consumo de carne de
animales MG no implica ningún riesgo para la salud humana.
La seguridad de los alimentos MG depende del transgén y su
producto, así como del fenotipo resultante. Los aspectos
éticos y de protección animal demandan la
generación de peces sanos. La seguridad ambiental requiere
de medidas eficientes de control biológico para minimizar
los posibles daños al ecosistema por la liberación de
peces MG. Los nuevos desarrollos en el campo de la resistencia a
enfermedades, serán beneficiosos para la producción y
el medio ambiente en caso de escape de peces MG. Debido a que la
acuicultura se aplica tanto a especies de agua dulce como marinas,
es posible su desarrollo en la mayor parte de los países del
mundo. Para evitar fracasos en las transferencias
tecnológicas, es importante adaptarse a las condiciones
regionales y locales, mediante la elaboración de proyectos
de colaboración capaces de desarrollar la capacidad de
implementación de la tecnología.
Palabras claves: acuicultura, peces, seguridad,
transgénico
Introduction
The FAO statistics for worldwide sea food production shows that
fish farming represents about 15% of the worldwide catch from
fisheries and is estimated to pass 20% by the year 2000 [1].
Currently, the maximal sustainable catch of wild fish has already
been reached, while an increase in seafood production can be only
gained through aquaculture. Increased production efficiency in
aquaculture will be acquired through genetic improvement of the
production species by selection breeding and, potentially, gene
transfer technology.
Fish farming in ponds and dams is known from China for 5000
years and from Europe, for around 1000 years. The first examples of
trout farming came just before the World War I and the Norwegian
salmon farming in net pens came in the seventies. A program for
selection breeding coordinated at the national level and developed
approximately ten years later was the breakthrough [2]. Six
generations of selection breeding have resulted in a Norwegian
salmon more than 50% bigger than its ancestors in the rivers of
Norway. The Institute for Aquacultural Research (AKVAFORSK) has
successfully implemented the Norwegian salmon breeding program to
tilapia farming in the Philippines and carp farming in India. The
history of farmed salmon makes it one of the latest domesticated
species together with other few farm fish species, although fish
culture as a general phenomenon has been known for thousands of
years.
A lot of effort is now made to domesticate novel species for the
benefit of aquaculture and the global demand for an increased food
production. Some 200 species are being cultivated world wide,
including 110 fish species, 25 crustaceans, 50 mollusks and 8
algae, but 80% of the world aquacultural production is derived from
a dozen species [1]. In order to achieve maximal improvement in new
breeding programs for aquatic organisms, genetic engineering and
genetically modified organisms (GMO) offer new possibilities. The
objective of this paper is to discuss technical, environmental and
management considerations of using GMOs in aquaculture.
Technical aspects
Genetic improvement of fish by gene transfer
The development of recombinant DNA technology has allowed to
move DNA from one species to another, regardless of the category of
organism. Thus, a plant, an animal or microorganisms like bacteria
or even a virus can be either donor or recipient of genetic
information encoded in the genome.
An organism receiving a new gene into its genome is called a GMO
or transgenic organism. The transgene can originate from a
phylogenitically distant organism or from the same species.
Nowadays this technological development makes possible the
improvement of fish and other aquatic organisms [3-8].
There are various methods for achieving gene transfer to fish.
The most common and so far the most successful is microinjection.
For microinjection of DNA, a capillary pipette with a tip 0.005 mm
in diameter is used for injecting 0.00000025 mL of a DNA solution
into fertilized fish eggs. The development of embryonic stem cells
and somatic cell cloning will allow qualitative and quantitative
predetermined gene insertion through homologous recombination
(today, researchers work only on mammals, but recent reports
suggest the introduction to other species including fish in a near
future). Another improvement regarding gene transfer efficiency to
fish involves complexing transgenes with synthetic NLS peptides
mediating active nuclear import [9].
Most recent reports on transgenic production of fish species
describe the transfer of "all fish" gene constructs, with a general
concern of utilizing DNA sequences derived from the same, or
closely related species. In such cases, truly new gene sequences
are not added to the genome, but the copy number of the already
existing genes is rather increased. However, changes are often made
to obtain an altered control of gene expression by fusing a
non-typical promoter to the coding region of the gene.
This technology however still has several major constrains: (i)
low efficiency generation of transgenics in some species, (ii) need
for species of close life cycle in the laboratory (iii) low
precision regarding copy numbers and integration site, and (iv)
need to identify, isolate and characterize the genes to transfer.
What species and what traits?
A number of species are in focus for gene transfer experiments
and can be divided into two main groups: animals used in
aquaculture [3-8] and model fish used in basic research [7, 10].
Among the major food fish species are carp (Cyprinus sp.),
tilapia (Oreochromis sp.), salmon (Salmo sp.,
Onchorryncus sp.) and channel catfish (Ictalurus
punctatus), while zebrafish (Danio rerio), medaka
(Oryzias latipes) and goldfish (Carassius auratus)
are used in basic research. In addition to food production and
research, carp and goldfish are widely used as ornamental fish.
Other fish sea food species important in aquaculture such as,
mollusks, shellfish and shrimps are now getting into the focus for
research on gene transfer-mediated improvements [11].
In the classical breeding programs, economically important
traits like growth rate and food conversion efficiency have been
top-ranked, aiming at an increased food production. Several other
traits of economical importance have not been possible to improve
with selection breeding. The pink color of salmon is still achieved
by adding synthetic carotenoids to the food pellets. The lack of
disease resistance, which made the heavy use of antibiotics
necessary until just a few years ago is now combated through the
development of new vaccination regimes.
Gene transfer technology currently applies only to single copy,
or few copy gene traits. Secondly, the candidate genes must be
characterized at the molecular level. Only a limited number of gene
traits fulfills both criteria and thus is applicable to genetic
engineering for breeding purposes, which makes necessary the
performance of further studies on genes controlling disease
resistance, aggression, flesh color, etc.
Growth control mediated by growth hormone (GH; somatotropin)
belongs to the best understood area of vertebrate physiology. The
success in the early eighties with the transfer of human and rat GH
genes to fertilized mouse eggs, which resulted in fast-growing
transgenic mice has since then stimulated many groups to try to
achieve genetic improvements of different farm animals by genetic
engineering. Several laboratories now have GM fish with increased
growth performance caused by extra copies of GH gene [8].
Transgenics with up to 30 times higher growth performance compared
to the average of nontransgenic siblings have been reported [6,
12]. However, to efficiently manipulate the process of growth, it
is necessary to characterize at the molecular level the mechanisms
involved in growth control to determine which genes to manipulate
and the expression levels to achieve growth acceleration for each
specie.
Freeze resistance achieved through antifreeze protein (AFP) is
another trait in focus of research efforts. AFP genes have been
transferred from the genome of the ocean pout (Macrozoarces
americanus) to salmon and goldfish with the aim of introducing
freeze resistance [6]. Recently, fish AFP genes have also been
transferred to plant species in order to make them resistant to
freezing.
There are two reasons for developing a sterile fish. Onset of
sexual maturation with gonad development results in loss of both
weight and meat quality in salmons. Secondly, sterile escaped farm
fish cannot reproduce in the wild, and thus represents an efficient
biological containment. Sterile fish is today achieved through
chromosome manipulation giving rise to triploid, often monosex,
fish. An attempt in making transgenic sterile fish is to antagonize
the production of the chief sex gonadotropin-releasing hormone
(GnRH) by the transfer of special anti-GnRH genes [13]. Other ideas
of introducing biological barriers against uncontrolled breeding
are discussed below.
Recent applications of gene transfer technology in fish have
focused on the development of "living biomarkers" for detecting
water pollutants and other environmental xenobiotics [7].
One special case of GM animals is that of bioreactors because
the animals are used exclusively for the production of a certain
protein. Fish have been suggested to be a candidate of this
category of organisms along with mammals and plants [7].
Environmental issues
Are GM fish safe to eat?
GM fish as such do not represent any health hazard. Of major
importance for health risk evaluation is the character of the gene,
the protein it encodes and the resulting phenotype. In addition, it
is important to assure that the insertion of a novel gene does not
affect an endogenous gene or has no other pleiotropic effects
[14].
Most of the existing GM fish prototypes have received extra
copies of their GH genes, resulting in only moderately elevated
levels of circulating GH. This hormone is a protein that is
degraded along with all other food proteins. Meat from fish
transgenic for GH is regarded as fully safe for human consumption.
Using the principle of "substantial equivalence", recent
experiments with GM fast-growing tilapia demonstrated that eating
GM meat is safe [15]. In addition, food quality control concerns
taste, appearance, color, texture and other parameters important
for a commercial success, but not necessarily for health safety.
Finally, consumers decide if they accept GM food or not.
Natural ecosystems
It is generally accepted that undeliberated release of farmed
fish into natural ecosystems should be prevented, especially if the
fish is genetically modified. Physical containment of fish farms
has proven to be efficient only in land-based plants. Net pens in
the sea are more prone to accidents because of extreme weather
conditions, and in several occasions accidental release of a large
number of farmed salmon has been reported. In the present, farmed
salmons of many norwegian rivers reproduce and compete with the
local strains, although they are generally less adapted (Hindar,
1996).
Transgenic fast-growing tilapia (line F70) retain many
characteristics of the parental O. hornorum hybrid,
including disease resistance and behavioral parameters [15-17].
This transgenic line growths 60-80% faster when compared to
non-transgenic lines depending on culture conditions [17].
Metabolic and food conversion efficiency is also better in
transgenic tilapia [16]. Transgenic tilapia require more energy
production to sustain a higher growth speed and this process is
more efficient than in wild-type tilapia with a food conversion
efficiency 3 times higher than that of wild-type tilapia [16].
After completing the evaluations required by Cuban authorities,
these tilapia are considered as safe for culture under controlled
conditions [18]. The introduction of this line into national
aquaculture has been estimated to produce important savings for the
industry (R Martínez, 1998).
Management: biosafety and risk assessment
It is generally accepted that time from basic research to
commercial applications is short in modern biotechnology.
Supporting this statement, there already exists a commercial
transgenic fast-growing AquAdvantage™ Atlantic salmon that in
about two years will be marketed worldwide by the US Company A/F
Protein Inc. (Aqua Bounty Farms, Waltham, MA, USA). Plans to
introduce it in Scottish fish farms have recently caused debate in
Europe. The establishment of a homozygous line of GM Atlantic
salmon takes a minimum of 13 years from the microinjection event
until the GM fish can be introduced into a breeding program [19].
For species like tilapia and carp, which have shorter life cycles,
this period is also shorter. Finally, as previously discussed,
before production can begin, the approval from the authorities with
regards to environmental and health safety is necessary. In Cuba,
transgenic fast-growing tilapia are commercially available for
local aquaculture and have been incorporated into the national
tilapia genetic program by the Ministry of Fisheries.
In addition to practical restrictions and limitations, general
ethical and animal protection concern demand animal health effects
of the transgene and its product to be adequately addressed before
any commercial fish farming can be initiated. If animal health is
not negatively affected by the transgene or its product, it can be
argued as an indication that GM fish is not hazardous for human
consumption [20]. In the case of fast-growing GH-transformed fish,
symptoms similar to acromegaly are seen in some of the animals
showing the highest levels of growth enhancement although the
general impression at present is that the majority of transgenics
are healthy.
Risk assessment of GMOs is given priority both by national and
international bodies engaged in biotechnology development [19,
21-25]. In August 1994, the First International Symposium on
Sustainable Fish Farming was held in Oslo, Norway [5]. During this
conference "The Holmenkollen guidelines for sustainable industrial
fish farming" were discussed and approved. Recommendation
Nr. 14 stated that "the farming of transgenic or other
genetically manipulated fish should not be undertaken until safety
and ethical criteria be established". The definition of GMO by the
International Council for Exploration of the Sea (ICES) includes
both transgenics and fish with modified chromosome sets, but not
fish improved by selection breeding. In the discussions, biological
containment regimes are suggested as an important complement to the
physical barriers.
In Cuba, to conduct the studies outside the laboratory, an ad
hoc committee meeting devoted to the analysis of the conditions
to release transgenic tilapia with accelerated growth was held
[18]. This committee concluded that, under the conditions found in
Cuba, little or no effect on natural populations would occur as a
result of accidental escape of these transgenic tilapia. Most of
the fresh water fish species currently found in the country have
been introduced and natural populations are represented only by few
species already in contact with introduced exotic tilapia species.
As evaluated by González-Sansón and Aguilar [26], for
example, the principal effect of the introduction in the Cuban
coastal lagoons of tilapia (O. aureus) was to
accelerate the fluxes of energy and matter. Tilapia consumes algae
which excrete partially digested therefore "pelletizing" the algae
mass of the lagoon and accelerating its incorporation into the
detritus. Because the most abundant species in the lagoon have
different feeding habits, the situation in the lagoon could be very
similar to the situation in polyculture.
Nevertheless, the committee recommended to follow the final
draft of the "Performance Standards for Safely Conducting Research
with Genetically Modified Fish and Shellfish" (documents Nr. 95-01
and 95-02) prepared and released by The US Department of
Agriculture, the Agricultural Biotechnology Research Advisory
Committee, and the Working Group on Aquatic Biotechnology and
Environmental Safety. These standards are accompanied by flowcharts
that guide the assessment pathway in a way that allows to consider
and implement the necessary measures to safely conduct the
experiments with transgenic fish for accumulating the data needed
to fully characterize these new fish strains.
Biological containment is well known from biosafety restrictions
of microorganisms used in recombinant DNA research and industrial
applications. These often include mutations in genes of metabolic
pathways, making the organism depend on the missing metabolite
supplemented in the growth medium. Biological containment in the
context of aquaculture includes sterile triploid fish or sterile
transgenic fish carrying anti-fertility genes tailor-made into
their genomes [13, 27]. In addition to the establishment and
reproduction in wild biotopes, escaped salmons have spread disease
to wild populations in several cases. This problem is not avoided
by fish sterility. Therefore, why introduction of suicide genes
that allow survival only in capture has been suggested. Triploid
fish technology is a simple cost-effective method used in many
countries to make sterile fish. The disadvantage is that every egg
must be treated and that the extra set of chromosomes also
represents a cellular content of nucleic acids 30% higher when
compared to normal fish. One EU Biotechnology research project,
"Biological containment of transgenic fish and risk assessment of
inter-species gene transfer" aims at making GM sterile fish by
expressing anti-fertility genes antagonizing the production and
action of the chief sex GnRH [13]. The advantage would be that
sterility becomes a stable inheritable trait, and only selected
brood stock animals are rendered fertile through hormone therapy.
These GM sterile fish would also carry the normal chromosome number
and DNA content.
Risk assessment in cases of deliberate or accidental release of
transgenic fish depends on a number of factors: (i) the species
released and the biotope it is released into, (ii) the character of
the transgene and the new phenotype, (iii) the general fitness of
the GMOs versus wild populations, and finally, (iv) the number of
released GM fish, which is an important factor. There exists quite
a wide experience from the introduction of exotic species in the
environment, which is helpful for making risk assessment of the
GMOs. Every new GMO could be regarded as a novel exotic species.
However, it does not prevent that a simple rule be followed. Since
there is no information about the new performance qualities of GMO
and since this knowledge is important to get, it is advised to gain
it with the "precautions principle" in mind. This means a "case by
case & step by step" procedure, starting with physically
contained testing in laboratories and moving to field tests via
small scale and intermediate scale to large scale. "Case by case"
represents the pre-calculation that can be made from evaluating the
aforementioned factors i-iv. Evaluation at each step will tell if
moving to next is acceptable.
International research collaboration and sustainable
development
According to Agenda 21 chapter 16, it will be of great
importance a broad international research collaboration at all
levels of research, from molecular characterization of genes of
quantitative trait loci (QTL) to environmental risk assessment
experiments. This will allow biotechnology to be used in support of
a sustainable development, giving the necessary concern for the
environment. Recent identification and application of polymorphic
fish microsatellites have proved valuable genetic markers with
regard to gene mapping, population genetic analysis and individual
identification [28].
In several areas ranging from fish culture economy to basic
research in biology and medicine, transgenic fish technology can be
foreseen to play an important role in the future (Table).
Table. What are the potentials for transgenic fish? (modified
from reference nr. 3).
I. Improve economy of fish culture
- increase growth rate
- increase overall size
- increase food conversion efficiencies
- utilize low cost diets (carbohydrates versus
protein)
- improve cold tolerance/freeze resistance
- improve disease resistance
- increase brood stock fecundity
- control smolting and reproduction
- reduce aggression
II. Tailor-made fish for the market
- flesh color, flavor and texture
- fatty acid composition
III. Fish as bioreactors
- production of commercially useful compounds
IV. Basic research
Up to now, improvement of growth in GM fish is the most
successful way of genetically modifying an economical trait,
achieved in several fish species in laboratories from both
developed and developing countries. A growing concern to avoid
farmed fish (both GM and non-GM) to escape and compete out wild
strains now calls for sterile fish unable to reproduce and
interbreed with wild populations. Only a few years ago disease
problems forced the Norwegian salmon farming industry to use large
amounts of antibiotics. The development of new fish vaccines has
largely solved the problem at present. The risk of disease together
with the cost of vaccination makes the development of disease
resistance through gene transfer a high priority research strategy.
Using transgenesis for an increased food production and a
sustainable development, a lot of investment must be made in the
necessary basic and applied research needed for the understanding
of how complex traits are controlled by single genes. In addition,
this research must be carried out based on a true international
collaboration so that basic competence can be developed in many
countries to use the technology, and the technology can be
developed according to the needs and socio-economical situation of
the user nation or region.
Acknowledgements
We wish to thank our staff for fruitful discussions. This paper
was updated after Aleström [29].
References
1. Sasson A. Marine biotechnology today: an overview
(achievements, challenges and prospects). The First Indonesian
Seminar on Marine Biotechnology. Gedung Widya Graha LIPI, Jakarta,
14-15 October 1998.
2. Gjedrem T, Gjerde B, Refstie T. A review of quantitative
genetic research in salmonids at AKVAFORSK. Proceedings at the
Second International Conference on Quantitative Qenetics, Sinauer,
Sunderland MA, 1988; 527-35.
3. Fletcher GL and Davies PL. Transgenic fish for aquaculture.
Genetic Engineering 1991;13:331-71.
4. Powers DA, Chen TT, Dunham RA. Biotechnology of aquatic
animals: a new frontier with implications for both basic and
applied research. Biology International 1993;28:17-25.
5. Aleström P. Genetic engineering in aquaculture. In:
Proceedings from the First International Symposium on Sustainable
Fish Farming; 1994 Avg 28-31; Oslo, Norway. Balkema: Reinertsen
& Haaland; 1995. p.125-30.
6. Hew CL, Fletcher GL, Davies PL. Transgenic salmon: tailoring
the genome for food production. Journal of Fish Biology
1995;47:1-19.
7. Chen TT, Lu J-K. Transgenic fish technology: basic principles
and their application in basic and applied research. In: De la
Fuente J and Castro FO, editors. Gene transfer in aquatic
organisms. Austin (Texas): RG Landes Company and Germany:
Springer-Verlag; 1998. p.45-73.
8. de la Fuente J. Gene transfer in aquatic organisms in the
framework of modern biotechnology. In: De la Fuente J, Castro FO,
editors. Gene transfer in aquatic organisms. Austin(Texas): RG
Landes Company and Germany: Springer-Verlag; 1998. p.1-15.
9. Collas P, Aleström P. Nuclear localization signals
enhance germline transmission of a transgene in zebrafish. Trans
Res 1998;7:303-9.
10. Aleström P, Husebye H, Kavumpurath S, Kisen G.
Zebrafish, a vertebrate model for transgene expression and
biological function. Animal Biotechnology 1994; 5:147-54.
11. Gómez-Chiarri M, Smith GJ, de la Fuente J, Powers DA.
Gene transfer in shellfish and algae. In: De la Fuente J, Castro
FO, editors. Gene transfer in aquatic organisms. Austin(Texas): RG
Landes Company and Germany: Springer-Verlag; 1998. p.107-25.
12. Devlin RH, Yesaki TY, Donaldson EM, Du SJ, Hew CL.
Production of germline transgenic Pacific salmonids with
dramatically increased growth performance. Can J Fish Aquat Sci
1995;52:1376-84.
13. Aleström P, Kisen G, Klungland H, Andersen Ø.
Fish gonadotropin-releasing hormone gene and molecular approaches
for control of sexual maturation - Development of a transgenic fish
model. Molecular Marine Biology and Biotechnology 1992;1:376-9.
14. OECD 1992 - Aquatic Biotechnology and Food Safety. ISBN
92-64-14063-8.
15. Guillén I, Berlanga J, Valenzuela C, Morales A,
Toledo J, Estrada MP, et al. Safety evaluation of transgenic
tilapia with accelerated growth. Mar Biotechnol In press 1999.
16. de la Fuente J, Guillén I, Martínez I, Estrada
MP. The control of the process of growth in tilapia: basic research
and applications. Biomolecular Engineering In press 1999.
17. de la Fuente J, Martínez R, Guillén I, Estrada
MP, Lleonart R. Gene transfer in tilapia for accelerated growth:
from the laboratory to the consumer. In: De la Fuente J, Castro FO,
editors. Gene transfer in Aquatic Organisms. Berlin:
Springer-Verlag and Georgetown: Landes Biocience; 1998.
p.83-106.
18. de la Fuente J, Hernández O, Martínez R,
Guillén I, Estrada MP, Leonart R. Generation,
characterization and risk assessment of transgenic tilapia with
accelerated growth. Biotecnología Aplicada
1996;13:221-30.
19. OECD 1993 - Environmental Impacts of Aquaculture using
Aquatic Organisms Derived through Modern Biotechnology. ISBN
92-64-14666-0.
20. Berkowitz DB, Kryspin-Sørensen I. Transgenic fish:
safe to eat? Bio/Technology 1994;12:247-51.
21. Hindar K. Genetically engineered fish and their possible
environmental impact. NINA Oppdragsmelding 1993; 215:1-48.
22. Pandian TJ, Marian LH. Problems and prospects of transgenic
fish production. Current Science 1994;66:635-49.
23. USDA ABRAC 1995, USDA Office of Agricultural Biotechnology,
Agricultural Biotechnology Research Advisory Committee. Performance
Standards for Safely Conducting Research with Genetically Modified
Fish and Shellfish.
24. International Council for Exploration of the Sea (ICES)
Working Group of the Application of Genetics in Fisheries and
Mariculture (WGAGFM) 1996. Chairman J. Mork, fax. +47-73591597,
E-mail jarle.mork@vm.unit.no
25. Knibb W. Negligible ecological risk is predicted for low
level releases of genetically engineered and modified marine fish.
3rd International Marine Biotechnology Conference; 1994 Aug;
Tromso, Norway. Tromso: Tromso University; 1994.
26. González-Sansón G, Aguilar C. Ecología
de las lagunas costeras de la región suroriental de Cuba.
Revista Investigaciones Marinas 1984;V(1):127-71.
27. Donaldson E, Devlin RH, Solar IH, Piferrer F. The
reproductive containment of genetically altered salmonids. In:
Cloud JC, Thorgaard GH, editors. New York: Plenum Press; 1993.
p.113-29.
28. Estrada MP, Portal O, de la Fuente J. Variation in
microsatellite sequences between tilapia belonging to the
Oreochromis genera. Mar Biotechnol. In press 1999.
29. Aleström P. Peces modificados genéticamente en
la acuicultura del futuro: Consideraciones técnicas,
ambientales y de manejo. En: Komen J, Falconi C, Hernández
H, editores. Transformación de las prioridades en programas
viables. Actas del seminario de política
biotecnológica agrícola para América Latina;
1996 Oct 6-8, Perú. Haya/México (DF): Intermediary
Biotechnology Service/CamBio Tec; 1998. p.92-7.
Received in December, 1998. Accepted for publication in
January, 1999.
Copyright 1999 Elfos Scientiae
|