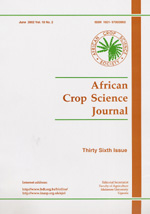
|
African Crop Science Journal
African Crop Science Society
ISSN: 1021-9730 EISSN: 2072-6589
Vol. 8, Num. 3, 2000, pp. 213-222
|
African Crop Science Journal, Vol. 8. No. 3, pp. 213-222
African Crop Science Journal, Vol. 8. No. 3, pp. 213-222
TRANGRESSIVE SEGREGATION FOR RESISTANCE IN WHEAT TO SEPTORIA TRITICI BLOTCH
P.F. ARAMA, J.E. PARLEVLIET1 and C.H. VAN SILFHOUT2
Kenya Agricultural Research Institute, National Plant Breeding
Research Centre, P.O. Njoro, Kenya 1Wageningen Agricultural University, Department of Plant Breeding, 6700 AJ, Wageningen, The Netherlands 2Research Institute for Plant Protection (IPO-DLO), P.O. Box 9060, 6700 GW, Wageningen, The Netherlands
(Received 5 March, 1998; accepted 14 March, 2000)
Code Number: CS00023
INTRODUCTION
Septoria tritici, the causal organism of septoria tritici
blotch (STB), is at present the second most important wheat pathogen after yellow
rust (Puccinia striiformis) in reducing yields in Kenya. The disease
is also of major importance in the highlands of Ethiopia and Tanzania, in the
coastal areas of the Mediterranean, in South America, in Australia and in Western
Europe (Saari and Wilcoxon, 1974; Rajaram and Dubin, 1977). A crop failure due
to blotch was reported from Kenya as early as the mid twenties by Burton (1927)
and efforts were made in the sixties to identify sources of resistance (Saari
and Wilcoxson, 1974). A Regional Disease and Insect Screening Nursery (RDISN)
was subsequently established. Some of the best sources of resistance to S.
tritici in the 1971-1972 nurseries were Kenya wheat lines selected in Ethiopia
(Pinto, 1972) and submitted for regional testing in the RDISN.
After this initial work in Kenya, little was done on STB over
the next two decades. This was probably due to the importance of stem rust (Puccinia
graminis) in the 1970s and that of yellow rust (Puccinia striiformis)
in the 1980s (Danial, pers. comm.). To improve stem rust resistance and yielding
capacity, early maturing semi-dwarf lines from the International Maize and Wheat
Improvement Centre (CIMMYT), Mexico were introduced into the breeding programme.
Because of the relationship between plant stature, maturity class and resistance
to STB (Danon et al., 1982; Eyal et al., 1983; Arama et al.,
1994) the more STB resistant germplasm was unconsciously discarded. Incidences
of STB increased gradually but was initially unnoticed. Favourable weather conditions
prevailing in Kenya in the 1985 and 1986 growing seasons led to severe STB epidemics
and a renewed interest in STB resistance.
Most of the high yielding wheat cultivars grown in Kenya today
are quite susceptible to STB. Cultivation of resistant cultivars is the economically
most feasible control measure for many wheat diseases. Undoubtedly, most breeders
and plant pathologists want a form of resistance that keeps its effectiveness
over time, and which is easy to transfer across genotypes, easy to identify
in segregating progenies and effective under disease conducive conditions. However,
germplasm resistant to STB is rather scarce, and little is known about the types
of resistance and the mode of inheritance (Eyal, 1981).
Conflicting reports are found in the literature regarding the
nature of genetic resistance to STB. These range from simple Mendelian genetics
to complex quantitative inheritance patterns. Mackie (1929) found, by analysing
F2 populations, that a single recessive gene provided resistance in an unidentified cultivar. Single dominant genes for resistance have been reported to be present in Lerma50 and P14 (Narvarez and Caldwell, 1957), Bulgaria 88 (Rillo and Caldwell, 1966), Veranopolis (Rosielle and Brown, 1979; Wilson, 1979), Carifen 12 (Lee
and Gough, 1984), Vilmorin (Gough and Smith, 1985) and IAS20/#567.1 (Jlibene,
1990). Two to three dominant genes have been found to confer resistance in Thornbird
(Jlibene, 1990).
Wilson (1985), in evaluating 28 sources of STB resistance found
that a single dominant gene was the most common type of genetic resistance.
However, there were some exceptions including duplicate dominant, single incomplete
dominant models, and for the cultivar Seabreeze, a two recessive gene model
was suggested. In other studies Camacho-Casas et al. (1995) reported
that additive and dominance effects were responsible for the resistance to STB
in II50-18/VGDWF/3/PMF. Wilson (1985) proposed three different genes conferring
resistance to STB. These were designated Slb1, Slb2 and Slb3 for the genes in
Bulgaria 88, Veranopolis and Israel 493, respectively. Van Ginkel (1986) suggested
that the search for single gene resistance to STB may be ineffective because
of the presence of modifier genes, lack of discrete classes in segregating populations,
evidence of transgressive segregation, disagreements on where to place the dividing
border line for segregation between resistance and susceptibility and environmental
influence. Failure to transfer satisfactory levels of resistance to septoria
leaf blotch was also noted by Eyal et al. (1987) who suggested the presence
of modifying genes that affect the expression of dominant genes for resistance.
In durum wheat, Van Ginkel and Scharen (1987) reported that resistance to Septoria
tritici blotch was explained by models involving additive and dominant gene
effects and that the additive gene effects were more important than dominant
gene effects. Epistatic gene effects were of minimal importance.
The objective of this experiment was to study the quantitative
inheritance of adult plant resistance of bread wheat to S. tritici.
MATERIALS AND METHODS
Fifty seven bread wheat cultivars were evaluated for their
resistance to natural inoculum of the S. tritici populations in Njoro
(2160 m) and Timau (2640 m) in 1988 and 1989. After correcting the disease severity
for maturity and tallness, 14 cultivars, which ranged from low to high levels
of resistance to STB, were selected (Table 1). A single ear of each cultivar
was harvested and planted in a 2 m row. At the heading stage some ears in each
row were bagged individually to ensure complete selfing. This was repeated in
1990 and 1991. Cultivars were grouped as early and late maturing. There were
seven early maturing and six late maturing entries. The cultivar 343 (TRAP#1*2//ERPS/RUSO),
a medium maturing cultivar, was included in both groups (Table 1).
TABLE 1. Pedigrees of parents used in the study of inheritance
of resistance to Septoria tritici blotch of wheat |
Code |
Pedigree/Name |
Early maturing |
396 |
BOWS/VEES |
343 |
TRAP#1*2//ERPS/RUSO |
327 |
RPB.1468/NAC//DOVES |
303 |
Clement (CNO-INIA*LFN/TOB*KI.PERAF) |
287 |
Frontatch (FRONTANA/K58/NEWTHATCH) |
244 |
HAHNS*/PRLS |
127 |
CMH79A.307/BOWS |
001 |
BUCS/BJYS |
Late maturing |
343 |
TRAP#1*2//ERPS/RUSO |
301 |
Jupateco-Alondra |
282 |
Finks |
279 |
Kenya Sungura (IDAHO 1877.NRBJx11-53-370=MORRIS) |
267 |
YAP/BJYS |
106 |
Ning 8331 |
019 |
Milan (VS73.600/MRLS/3/BOWS//YR/TFRS |
The entries were planted four times at an interval of 10 days
beginning 23 September 1991. Half-diallel crosses were made within each group.
F1 progenies and F2 generations realised were planted widely spaced in 2 rows, 4 m long in 1992. Some crosses were discarded in F3 because they were found to have been mixed up. Only 36 crosses were harvested in the F3. From each cross about 100 ears were randomly and individually harvested. The ears were planted n rows at a wide spacing to raise F4 populations. This was repeated to raise the F5. Three plants from each row were harvested and threshed separately.
In 1995 the seeds of three plants from an F5 line were planted in three rows to make F6 plots. Rows were planted 1.5 m long, 20 cm apart. The two parents of the cross were planted in 3 rows after every 20 plots. Because
of infrequent rainfall in the months of June and July, irrigation was provided
twice a week.
Inoculum preparation and inoculations. The isolate IPO93001
(previously sampled from Njoro) was selected for the inoculations. An infected
leaf segment was attached to a glass slide and placed in a petri dish fitted
on the bottom with filter paper saturated with sterile water. The petri dish
cover was replaced to provide a moist environment for 4 hrs. It was then transferred
to a laminar-flow clean air cabinet bench. Oozing pycnidia were located under
the stereoscopic microscope. With the help of a fine-pointed needle, sterilised
in a flame and cooled briefly, a single cirrus was picked and transferred to
Yeast-Malt Agar (YMA) (Eyal et al., 1987) medium in a petri dish. The
growing colony was spread on the medium and sub-cultured in fresh media for
multiplication.
The cultures were inoculated into 1 L Erlenmeyer flasks containing
1 L Yeast sucrose liquid medium (Eyal et al., 1987). Cultures were shaken
on a Lab-Line Orbit Shaker at 150 RPM for five days. The shaker was turned off
overnight to allow the spores to settle down. The liquid medium was carefully
decanted after which spores were re-suspended in distilled water and filtered
through a cloth filter. The spore concentration was determined and adjusted
to 1x106 spores/ml. Tween 20 surfactant was added into the inoculum just before inoculation. A CP15 knapsack sprayer was used for inoculations. The experiment
was inoculated four times at an interval of seven days beginning 30 days after
planting.
The heading date for each plot was noted and marked. Plants
in plots that had similar heading dates were observed at the same time. Five
main tillers were sampled at random from the middle row of each plot. Percentage
necrosis and percentage pycnidia coverage were visually assessed on the two
uppermost leaves.
As the irrigation was not uniform on all the plots the disease
spread was not expected to be uniform. Indeed the disease severity (DS) as measured
on the same genotypes (parents) did show clear variations. The mean percentage
pycnidia coverage of the two leaves after logit transformation, was therefore
corrected for within site variation based on 2-d- polynomials and fitted with
the SAS PROC GENMOD method (SAS., 1985) using the DS observed on the manyfold
replicated parents in the 36 crosses. The frequency distribution of the progenies
was calculated and grouped at 10% DS interval classes.
RESULTS
Testing of the various F6 wheat lines with isolate IPO93001
showed that there was clear transgressive segregation for DS in many of the
crosses. Transgressive segregation was observed for increased resistance, increased
susceptibility or both.
The extent of transgressive segregation varied among the crosses
(Table 2). Cross nrs. 17 and 18 had more than 50% of the population segregating
towards more resistance than the most resistant parent. On the other hand, the
cross nrs. 8, 10, 20, 21, 22, 23, 26, 27, 28, 30, 31 33, 35 and 36 showed over
50% segregation towards more susceptibility than the susceptible parent. There
was an increase in resistance in relation to the mid-parent (MP) values in five
out of the 36 crosses (Table 3). The greatest increase in resistance obtained
was 45% in relation to the mid-parent (MP) value from the cross 244 x 019. However,
most crosses showed a decrease in resistance compared to the MP-value. The highest
decrease of resistance obtained was 179.8% from the cross 303 x 001. The overall
mean MP value was 34.8% while the corresponding F6 mean was 47.7%,
a considerable increase in susceptibility. The standard deviations for F6
and the MP value were both 12.3. The correlation coefficient between F6
and MP-values was 0.44, while the correlation coefficient between the MP-values
and percentage change of the F6 from the MP-values was 0.73, both
being highly significant.
Table 2. Frequency distribution of F6 lines in 10
disease severity classes of 36 crosses obtained from 14 parents |
Cross |
Mean |
Disease severity class (percentage) |
0-10 |
11-20 |
21-30 |
31-40 |
41-50 |
51-60 |
61-70 |
71-80 |
81-90 |
100 |
1 |
F6 |
|
2 |
1 |
2 |
5 |
15 |
25a |
43a |
11 |
0 |
0 |
287b |
52.1 |
|
|
|
|
|
|
|
|
|
|
244b |
67.9 |
|
|
|
|
|
|
|
|
|
|
2 |
F6 |
|
0 |
1 |
3 |
8 |
7 |
19 |
25 |
38 |
5 |
0 |
327 |
50.6 |
|
|
|
|
|
|
|
|
|
|
244 |
67.9 |
|
|
|
|
|
|
|
|
|
|
3 |
F6 |
|
7 |
2 |
11 |
11 |
8 |
21 |
3 |
27 |
12 |
0 |
327 |
50.6 |
|
|
|
|
|
|
|
|
|
|
287 |
52.1 |
|
|
|
|
|
|
|
|
|
|
4 |
F6 |
|
4 |
2 |
5 |
6 |
3 |
7 |
1 |
19 |
1 |
0 |
301 |
72.9 |
|
|
|
|
|
|
|
|
|
|
279 |
29.3 |
|
|
|
|
|
|
|
|
|
|
5 |
F6 |
|
3 |
9 |
3 |
14 |
3 |
14 |
15 |
22 |
15 |
0 |
396 |
32.9 |
|
|
|
|
|
|
|
|
|
|
244 |
67.9 |
|
|
|
|
|
|
|
|
|
|
6 |
F6 |
|
1 |
2 |
6 |
9 |
20 |
12 |
6 |
21 |
4 |
0 |
343 |
30.4 |
|
|
|
|
|
|
|
|
|
|
244 |
67.9 |
|
|
|
|
|
|
|
|
|
|
7 |
F6 |
|
2 |
6 |
8 |
28 |
32 |
19 |
0 |
8 |
0 |
0 |
127 |
29.8 |
|
|
|
|
|
|
|
|
|
|
244 |
67.9 |
|
|
|
|
|
|
|
|
|
|
8 |
F6 |
|
1 |
2 |
2 |
7 |
7 |
11 |
2 |
33 |
42 |
0 |
396 |
32.9 |
|
|
|
|
|
|
|
|
|
|
287 |
52.1 |
|
|
|
|
|
|
|
|
|
|
9 |
F6 |
|
0 |
3 |
13 |
16 |
8 |
27 |
9 |
22 |
8 |
1 |
343 |
30.4 |
|
|
|
|
|
|
|
|
|
|
287 |
52.1 |
|
|
|
|
|
|
|
|
|
|
10 |
F6 |
|
4 |
3 |
12 |
7 |
19 |
12 |
41 |
16 |
0 |
|
327 |
50.6 |
|
|
|
|
|
|
|
|
|
|
396 |
32.9 |
|
|
|
|
|
|
|
|
|
|
11 |
F6 |
|
15 |
12 |
12 |
12 |
21 |
18 |
9 |
4 |
0 |
0 |
127 |
29.8 |
|
|
|
|
|
|
|
|
|
|
287 |
52.1 |
|
|
|
|
|
|
|
|
|
|
12 |
F6 |
|
6 |
10 |
13 |
17 |
5 |
22 |
6 |
17 |
2 |
0 |
244 |
67.9 |
|
|
|
|
|
|
|
|
|
|
001 |
13.2 |
|
|
|
|
|
|
|
|
|
|
13 |
F6 |
|
0 |
5 |
9 |
10 |
9 |
24 |
4 |
31 |
0 |
0 |
343 |
30.4 |
|
|
|
|
|
|
|
|
|
|
327 |
50.6 |
|
|
|
|
|
|
|
|
|
|
14 |
F6 |
|
10 |
8 |
12 |
10 |
2 |
12 |
4 |
18 |
15 |
0 |
327 |
50.6 |
|
|
|
|
|
|
|
|
|
|
127 |
29.8 |
|
|
|
|
|
|
|
|
|
|
15 |
F6 |
|
2 |
11 |
16 |
23 |
22 |
14 |
11 |
5 |
2 |
0 |
303 |
6.6 |
|
|
|
|
|
|
|
|
|
|
244 |
67.9 |
|
|
|
|
|
|
|
|
|
|
16 |
F6 |
|
0 |
9 |
7 |
20 |
6 |
19 |
8 |
25 |
0 |
0 |
301 |
72.9 |
|
|
|
|
|
|
|
|
|
|
106 |
1.4 |
|
|
|
|
|
|
|
|
|
|
17 |
F6 |
|
61 |
14 |
4 |
6 |
1 |
8 |
2 |
6 |
2 |
0 |
244 |
67.9 |
|
|
|
|
|
|
|
|
|
|
019 |
14.1 |
|
|
|
|
|
|
|
|
|
|
18 |
F6 |
|
43 |
16 |
6 |
3 |
3 |
5 |
0 |
21 |
9 |
0 |
267 |
41.5 |
|
|
|
|
|
|
|
|
|
|
279 |
29.3 |
|
|
|
|
|
|
|
|
|
|
19 |
F6 |
|
0 |
1 |
4 |
18 |
12 |
39 |
8 |
16 |
6 |
0 |
282 |
25.6 |
|
|
|
|
|
|
|
|
|
|
267 |
41.5 |
|
|
|
|
|
|
|
|
|
|
20 |
F6 |
|
0 |
1 |
6 |
3 |
4 |
7 |
7 |
28 |
42 |
7 |
327 |
50.6 |
|
|
|
|
|
|
|
|
|
|
001 |
13.2 |
|
|
|
|
|
|
|
|
|
|
21 |
F6 |
|
0 |
5 |
11 |
14 |
19 |
23 |
9 |
32 |
3 |
0 |
343 |
30.4 |
|
|
|
|
|
|
|
|
|
|
396 |
32.9 |
|
|
|
|
|
|
|
|
|
|
22 |
F6 |
|
7 |
9 |
14 |
18 |
21 |
17 |
14 |
11 |
1 |
0 |
127 |
29.8 |
|
|
|
|
|
|
|
|
|
|
396 |
32.9 |
|
|
|
|
|
|
|
|
|
|
23 |
F6 |
|
1 |
2 |
13 |
16 |
20 |
25 |
5 |
25 |
1 |
0 |
343 |
30.4 |
|
|
|
|
|
|
|
|
|
|
127 |
29.8 |
|
|
|
|
|
|
|
|
|
|
24 |
F6 |
|
8 |
8 |
13 |
21 |
15 |
14 |
9 |
3 |
1 |
0 |
303 |
6.6 |
|
|
|
|
|
|
|
|
|
|
287 |
52.1 |
|
|
|
|
|
|
|
|
|
|
25 |
F6 |
|
6 |
13 |
12 |
21 |
10 |
15 |
17 |
8 |
1 |
0 |
303 |
6.6 |
|
|
|
|
|
|
|
|
|
|
327 |
50.7 |
|
|
|
|
|
|
|
|
|
|
26 |
F6 |
|
2 |
1 |
11 |
19 |
10 |
11 |
4 |
20 |
6 |
0 |
282 |
25.6 |
|
|
|
|
|
|
|
|
|
|
343 |
30.4 |
|
|
|
|
|
|
|
|
|
|
27 |
F6 |
|
6 |
18 |
8 |
5 |
6 |
13 |
1 |
20 |
21 |
0 |
267 |
41.5 |
|
|
|
|
|
|
|
|
|
|
019 |
14.4 |
|
|
|
|
|
|
|
|
|
|
28 |
F6 |
|
6 |
4 |
6 |
7 |
4 |
21 |
4 |
25 |
16 |
0 |
282 |
25.6 |
|
|
|
|
|
|
|
|
|
|
279 |
29.3 |
|
|
|
|
|
|
|
|
|
|
29 |
F6 |
|
22 |
4 |
10 |
11 |
2 |
5 |
2 |
19 |
19 |
0 |
396 |
32.9 |
|
|
|
|
|
|
|
|
|
|
001 |
13.2 |
|
|
|
|
|
|
|
|
|
|
30 |
F6 |
|
0 |
2 |
7 |
15 |
3 |
21 |
6 |
42 |
5 |
0 |
343 |
30.4 |
|
|
|
|
|
|
|
|
|
|
001 |
13.2 |
|
|
|
|
|
|
|
|
|
|
31 |
F6 |
|
3 |
3 |
6 |
14 |
11 |
18 |
9 |
11 |
4 |
0 |
127 |
29.8 |
|
|
|
|
|
|
|
|
|
|
001 |
13.2 |
|
|
|
|
|
|
|
|
|
|
32 |
F6 |
|
22 |
8 |
5 |
10 |
3 |
10 |
3 |
5 |
1 |
0 |
282 |
25.6 |
|
|
|
|
|
|
|
|
|
|
019 |
14.4 |
|
|
|
|
|
|
|
|
|
|
33 |
F6 |
|
7 |
7 |
4 |
15 |
17 |
22 |
13 |
12 |
4 |
0 |
|
303 |
6.6 |
|
|
|
|
|
|
|
|
|
396 |
329 |
|
|
|
|
|
|
|
|
|
|
34 |
F6 |
|
28 |
21 |
29 |
11 |
4 |
1 |
1 |
0 |
0 |
0 |
|
303 |
6.6 |
|
|
|
|
|
|
|
|
|
127 |
298 |
|
|
|
|
|
|
|
|
|
|
35 |
F6 |
|
3 |
16 |
19 |
11 |
19 |
13 |
0 |
3 |
0 |
0 |
282 |
25.6 |
|
|
|
|
|
|
|
|
|
|
106 |
1.4 |
|
|
|
|
|
|
|
|
|
|
36 |
F6 |
|
29 |
15 |
15 |
15 |
12 |
6 |
6 |
4 |
0 |
0 |
303 |
6.6 |
|
|
|
|
|
|
|
|
|
|
001 |
13.2 |
|
|
|
|
|
|
|
|
|
|
aUnderlined and italic numbers indicate the disease
severity classes in which the parents occurred
bParents codes (Table 1) with their mean disease severity |
TABLE 3. Mean disease severity of parents (P1, P2), F6
and the midparent (MP), percentage decrease (-) or increase (+) in mean
disease severity in the F6 compared to the MP value, and the
range in the disease severity, where tr = trace, in the F6 of
36 crosses |
Cross |
P1 |
P2 |
F6 |
MP |
Change of F6 from MP, % |
287 x 244 |
52.1 |
67.9 |
56.9 |
60.0 |
-5.2 |
327 x 244 |
50.6 |
67.9 |
62.5 |
59.3 |
+5.4 |
327 x 287 |
50.6 |
52.1 |
53.7 |
51.4 |
+4.5 |
301 x 279 |
72.9 |
29.3 |
51.7 |
51.1 |
+1.2 |
396 x 244 |
32.9 |
67.9 |
56.3 |
50.4 |
+11.7 |
343 x 244 |
30.4 |
67.9 |
52.9 |
49.2 |
+7.5 |
127 x 244 |
29.8 |
67.9 |
42.4 |
48.9 |
-13.3 |
396 x 287 |
32.9 |
52.1 |
69.4 |
42.5 |
+63.3 |
343 x 287 |
30.4 |
52.1 |
50.1 |
42.3 |
+18.4 |
327 x 396 |
50.6 |
32.9 |
64.1 |
41.8 |
+53.3 |
127 x 287 |
29.8 |
52.1 |
36.8 |
41.0 |
-10.2 |
244 x 001 |
67.9 |
13.2 |
44.6 |
40.6 |
+9.9 |
343 x 327 |
30.4 |
50.6 |
53.9 |
40.5 |
+33.1 |
327 x 127 |
50.6 |
29.8 |
49.0 |
40.2 |
+21.9 |
303 x 244 |
6.6 |
67.9 |
41.5 |
37.3 |
+11.3 |
301 x 106 |
72.9 |
1.4 |
50.2 |
37.2 |
+34.9 |
244 x 019 |
67.9 |
14.1 |
19.8 |
36.0 |
-45.0 |
267 x 279 |
41.5 |
29.3 |
32.6 |
35.4 |
-7.9 |
282 x 267 |
25.6 |
41.5 |
54.4 |
33.6 |
+61.9 |
327 x 001 |
50.6 |
13.2 |
76.6 |
31.9 |
+140.1 |
343 x 396 |
30.4 |
32.9 |
53.4 |
31.7 |
+68.5 |
127 x 396 |
29.8 |
32.9 |
43.3 |
31.4 |
+37.9 |
343 x 127 |
30.4 |
29.8 |
50.7 |
30.1 |
+68.4 |
303 x 287 |
6.6 |
52.1 |
38.7 |
29.4 |
+31.6 |
303 x 327 |
6.6 |
50.6 |
42.0 |
28.6 |
+46.9 |
282 x 343 |
25.6 |
30.4 |
51.1 |
28.0 |
+82.5 |
267 x 019 |
41.5 |
14.1 |
51.1 |
27.8 |
+83.8 |
282 x 279 |
25.6 |
29.3 |
51.6 |
27.5 |
+87.6 |
396 x 001 |
32.9 |
13.2 |
46.2 |
23.1 |
+100.0 |
343 x 001 |
30.4 |
13.2 |
59.3 |
21.8 |
+172.0 |
127 x 001 |
29.8 |
13.2 |
49.8 |
21.5 |
+131.6 |
282 x 019 |
25.6 |
14.1 |
30.5 |
19.9 |
+53.3 |
303 x 396 |
6.6 |
32.9 |
47.8 |
19.8 |
+141.4 |
303 x 127 |
6.6 |
29.8 |
19.7 |
18.2 |
+8.2 |
282 x 106 |
25.6 |
1.4 |
34.6 |
13.5 |
+156.3 |
303 x 001 |
6.6 |
13.2 |
27.7 |
9.9 |
+179.8 |
Mean |
|
|
47.7 |
34.8 |
+51.3 |
Std. dev. |
|
|
12.3 |
12.3 |
|
DISCUSSION
Genetic studies in inheritance of resistance to STB have generally
been conducted on seedlings in the greenhouse and have been oriented towards
examining effects of simple Mendelian inheritance. However, in practical plant
breeding situations, selection is done in the field for an array of traits,
most of which are exposed in later stages of plant development and are quantitatively
inherited. Ballantyne (1985) and Camacho-Casas et al. (1995) reported
that plant reaction to S. tritici in F3 lines did not suggest simple
inheritance. Moreover, there have been reports of resistant cultivars being
obtained from parents of susceptible background (Shaner et al., 1975;
Shaner and Finney, 1982; Wallwork and Johnson, 1983; Lee and Shaner, 1985; Milus
and Line, 1986; Rose-Fricker et al., 1986; Schultz and Line, 1992; Poysa,
1993; Campbell and Wernsman, 1994; Campbell and White, 1995), suggesting that
resistance can result from a combination of genes that individually are ineffective
(epistatic effects).
The data from this study show that transgressive segregation
towards more resistance and/or more susceptibility to STB in wheat occurred
in most of the crosses. Because so many parents were involved, it suggests that
transgressive segregation should be obtained from many other crosses as well
and is not just an occasional phenomenon. The frequent occurrence of transgressive
segregants indicates the multigenic nature of resistance in the cultivars used.
As an illustration, Table 4 gives a half diallel crosses involving five parents,
from Table 2, with all F6s showing transgressive segregation. It
can be deduced that 287 and 327 must differ for at least two genes. In this
case recombination is possible and some F6 lines are either more
susceptible or resistant than the parents. But then 287 differs also from 396,
343 and 127 for at least two genes and that cannot be the same genes, otherwise
the transgression among the others cannot be explained. So with so many parents,
most showing transgression, the conclusion is that a fair number of genes are
involved.
TABLE 4. Mean disease severities (DS) and transgressive segregation
in a half diallel of crosses involving five parents |
Parent |
Parent Mean DS |
287 52.1 |
327 50.6 |
396 32.9 |
343 30.4 |
127 29.8 |
287 |
|
- |
+a |
+ |
+ |
+ |
327 |
|
|
- |
++ |
++ |
++ |
396 |
|
|
|
- |
++ |
++ |
343 |
|
|
|
|
- |
++ |
127 |
|
|
|
|
|
- |
a+ means some transgression; ++ means considerable
transgression |
Transgressive segregation for higher resistance to S. tritici
was demonstrated in progenies of a number of crosses. These transgressive segregants
clearly originated from a combination of genetic components from both parents
of each cross where the phenomenon was occurring. The highest increase in resistance
was obtained from the cross 244 x 019 (susceptible x resistant). Of the F6 population, 58% were more resistant than 019 (Table 2). This suggests that there are genes in the susceptible parent 244 that contribute to resistance. This is confirmed
from other crosses with 244 (crosses 1, 2, 5 and 7) where transgression beyond
the resistant parent occurs also. Moreover, it was observed that in most of
the crosses involving 244, the F6 means were almost equal to the MP values.
Another interesting increase in resistance was observed in the cross 287 x 244
(susceptible x susceptible). Pope (1968) hypothesised that genes controlled
functions in a sequence of events leading to resistance. In this case, each
gene alone has no effect, but high levels of resistance can be achieved when
the necessary combination of genes is produced by crossing.
The combination of quantitative resistance and transgressive
segregation is indicative of at least a few genes operating in an additive way.
More work need to be done to identify the resistance factors in the susceptible
cultivars 244 (HAHNS*/PRLS and 287 (Frontatch).
Out of the 36 crosses analysed, 31 crosses had F6 population
means higher than the mid-parent value, indicating greater susceptibility than
expected from the parental performance. It was observed that the crosses involving
the parents 001, 282 and 343 had most of the transgressive segregants towards
more susceptibility than the susceptible parent. It could be said that these
cultivars had a poor general combining ability. Their resistance being to a
fair extent of a non-additive nature (inter-locus interactions). Such cultivars
are of little use in breeding for resistance.
The high correlation between MP and the loss in resistance
is interesting. So, the higher the resistance of the parents, the more the F6
tended to less resistance, an observation of importance for breeders.
It is clearly shown that resistance to STB can be obtained
by crossing commercial wheat cultivars which are considered fairly to moderately
susceptible. This not only avoids introducing undesirable traits which are often
associated with resistant parents of exotic or non agronomic types but also
increases the probability of obtaining progenies with well adapted agronomic
traits suitable for cultivar development. Selection can then be done on resistant
transgressive segregants within the F6 population. This reduces the chances
of a single gene based resistance, generally vulnerable to adaptation by the
pathogen.
ACKNOWLEDGEMENTS
The trial discussed in this paper was supported by the Kenya
Agricultural Research Institute and by the Dutch Ministry for International
Cooperation (DGIS). The authors wish to thank the technical and support staff
at each participating research institutes for their role in trial execution
and data collection.
REFERENCES
- Arama, P.F., Parlevliet, J.E. and van Silfhout, C.H. 1994. Effect of plant
height and days to heading on the expression of resistance in Triticum
aestivum to Septoria tritici in Kenya. In: Proceedings of the
4th International workshop on: Septoria of Cereals. Arseniuk, E., Goral,
T. and Czembor, P. (Eds). pp. 153-157. IHAR, Radzikow, Poland.
- Ballantyne, B. 1985. Resistance to speckled leaf blotch of wheat in Southern
New South Wales. In: Septoria of Cereals. Proceedings of a Workshop.
Scharen, A.L. (Ed.), pp. 31-32. Bozeman, MT. USDA-ARS Publ. no.12.
- Burton, G.J.L. 1927. Report of Plant Breeder. Annual Report of the Department
of Agriculture, Kenya, 1926. pp. 158-171.
- Camacho-Casas, M.A., Kronstad, W.E. and Scharen, A.L. 1995. Septoria
tritici resistance and associations with agronomic traits in a wheat cross.
Crop Science 35:971-976.
- Campbell, K.G. and Wersman, E.A. 1994. Selection among haploid sporophytes
for resistance to black shank in tobacco. Crop Science 34:662-667.
- Campbell, K.W. and White, D.G. 1995. Inheritance of resistance to aspergillus
ear rot and aflatoxin in corn genotypes. Phytopathology 85:886-896.
- Danon, T., Sacks, J.M. and Eyal, Z. 1982. The relationship among plant stature,
maturity class, and susceptibility to septoria leaf blotch of wheat. Phytopathology
72:1037-1042.
- Eyal, Z. 1981. Integrated control of septoria diseases of wheat. Plant
Disease 65: 763-768.
- Eyal, Z., Wahl, I. and Prescott, J.M. 1983. Evaluation of germplasm response
to septoria leaf blotch of wheat. Euphytica 32:439-446.
- Eyal, Z., Scharen, A.L., Prescott, J.M. and van Ginkel, M. 1987. The septoria
diseases of wheat: Concepts and methods of disease management. Mexico D.F.:
CIMMYT. 46 pp.
- Gough, F.J. and Smith, E.L. 1985. A genetic analysis of Triticum aestivum
Vilmorin resistance to speckled leaf blotch and pyrenophora tan spot. In:
Septoria of Cereals. Proc. Workshop. Scharen, A.L. (Ed.), pp. 36. Bozeman,
MT. USDA-ARS Publ. No.12.
- Jlibene, M. 1990. Inheritance of resistance to septoria tritici blotch (Mycosphaerella
graminicola) in hexaploid wheat. Ph.D. Thesis, University of Missouri-Columbia.
86 pp.
- Lee, T.S. and Gough, F.J. 1984. Inheritance of septoria leaf blotch (Septoria
tritici) and pyrenophora tan spot (Puccinia tritici-repentis) resistance
in Triticum aestivum cv. Carifen 12. Plant Disease 68:848-851.
- Lee, T.S. and Shaner, G. 1985. Transgressive segregation of length of latent
period in crosses between slow-rusting wheat cultivars. Phytopathology
75:643-647.
- Mackie, W.W. 1929. Resistance to Septoria tritici in wheat. (Abstr.)
Phytopathology 19:1139-1140.
- Milus, E.A. and Line, R.F. 1986. Number of genes controlling high-temperature,
adult-plant resistance to stripe rust in wheat. Phytopathology 76:93-96.
- Narvarez, I. and Caldwell, R.M. 1957. Inheritance of resistance to leaf
blotch of wheat caused by Septoria tritici. Phytopathology 47:529-530.
- Pinto, F.F. 1972. Development of Septoria tritici in wheat and sources
of resistance in Ethiopia. (Cited by Saari and Wilcoxson, 1974).
- Pope, W.K. 1968. Interaction of minor genes for resistance to stripe rust
in wheat. In: Proceedings of the 3rd International Wheat Genetics Symposium.
pp. 251-257. Canberra.
- Poysa, V. 1993. Evaluation of tomato breeding lines resistant to bacterial
canker. Canadian Journal of Plant Pathology 15:301-304.
- Rajaram, S. and Dubin, H.J. 1977. Avoiding genetic vulnerability in semi-dwarf
wheats. Annual New York Academy of Science 287:243-254.
- Rillo, A.O. and Caldwell, R.M. 1966. Inheritance of resistance to Septoria
tritici in Triticum aestivum subsp. vulgare. Bulgaria 88.
(Abstr.). Phytopathology 56:597.
- Rose-Fricker, C.A., Meyer, W.A. and Kronstad, W.E. 1986. Inheritance of
resistance to stem rust (Puccinia graminis subsp. graminicola)
in six rye grass (Lolium perenne) crosses. Plant Disease 70:678-681.
- Rosielle, A.A. and Brown, A.G.P. 1979. Inheritance, heritability and breeding
behaviour of three sources of resistance to Septoria tritici in wheat.
Euphytica 28:285-392.
- Saari, E.E. and Wilcoxson, R.D. 1974. Plant disease situation of high yielding
dwarf wheats in Asia and Africa. Annual Review of Phytopathology 12:
49-68.
- SAS. 1985. Users guide: Basics, Versions Edition. SAS Institute Inc. Cary,
NC, USA. 1290 pp.
- Schultz, T.R. and Line, R.F. 1992. Identification and selection of F6
and F7 families of wheat for high-temperature, adult-plant resistance to stripe
rust using hill plots. Plant Disease 76:253-256.
- Shaner, G., Finney, R.E. and Patterson, F.L. 1975. Expression and effectiveness
of resistance in wheat to septoria leaf blotch. Phytopathology 65:761-766.
- Shaner, G. and Finney, R.E. 1982. Resistance in soft red winter wheat to
Mycosphaerella graminicola. Phytopathology 72:154-158.
- Van Ginkel, M. 1986. Inheritance of resistance in wheat to Septoria tritici.
Ph.D. Thesis, Montana State University. 102 pp.
- Van Ginkel, M. and Scharen, A.L. 1987. Generation mean analysis and heritabilities
of resistance to Septoria tritici in durum wheat. Phytopathology
77:1629-1633.
- Wallwork, H. and Johnson, R. 1983. Transgressive segregation for resistance
to yellow rust in wheat. Euphytica 33:123-132.
- Wilson, R.E. 1979. Resistance to Septoria tritici in two wheat cultivars
determined by two independent, single dominant genes. Australasian Plant
Pathology 8:16-18.
- Wilson, R.E. 1985. Inheritance of resistance to Septoria tritici
in wheat. In: Septoria of cereals. Proceedings of the Workshop.
Scharen, A.L. (Ed.), pp. 33-35. Bozeman, MT. USDA-ARS Publ. No.12
|