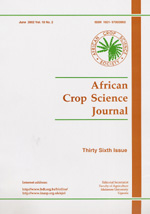
|
African Crop Science Journal
African Crop Science Society
ISSN: 1021-9730 EISSN: 2072-6589
Vol. 9, Num. 2, 2001, pp. 377-384
|
African Crop Science Journal, Vol. 9. No. 2, pp. 377-384
African Crop Science Journal, Vol. 9. No. 2, pp. 377-384
STUDIES ON GENETIC VARIATIONS IN A SORGHUM VARIETY IRRADIATED WITH COBALT 60 (CO60)
D.A. ABA, C.C. NWASIKE, M.YEYE and A.A. ZARIA
Plant Science Department, Institute for Agricultural Research, Ahmadu Bello University, Samaru, Zaria, Nigeria
(Recieved 10 May, 1999; accepted 8 June, 2000)
Code Number: CS01019
INTRODUCTION
Sorghum (Sorghum bicolor Moench) is a major staple food
in many parts of the world. It has very extensive use as a food crop in Africa,
Asia, and is a major feed grain in North America and South Africa (Pickett and
Oswalt, 1971). In areas where sorghum is used as a food crop the food recipes
in a given area have imposed severe restriction on the types of grain that may
be used in varietal development. In India, the preference is primarily for the
white, creamy white to yellow and pearly seeds types. In Africa, the variability
of choice is tremendous with the various types being the primary factor in food
preferences. As a feed grain in the United States, it is utilised as whole grain
(Pickett and Oswalt, 1971).
In Nigeria about 40 percent of the calories from all food stuffs
come from cereals and of this, sorghum contributes 50 percent, with almost all
the production coming from the Northern zone of the country (Oke, 1976). Sorghum
is a very versatile and hardy crop that is adapted to varying environments,
from the wet guinea savanna through to the vast dry sudan savanna and semi-
arid sahel zones in Nigeria. The sorghum growing areas in Nigeria lie between
latitudes 6° 30’N and 13° 55’N of the country.
Sorghum (Sorghum bicolar (L.) Moench), locally called
guinea corn is a very important crop in the savanna and derived savanna zones
of Nigeria, grown on about 6 million ha which cover about 9.7% of the world
sorghum growing areas. This relates to about 46% of the total land area devoted
to cereals production with a current estimated production of about 8 million
metric tonnnes (NAERLS, 1996; FAO/ICRISAT, 1996) in Nigeria. Of this amount,
3.12 million tonnes are consumed as human food and the remaining 0.88 million
tonnes is used for various industrial purposes, the most important being brewing
(Ikediobi, 1989, unpubli.).
In developing various types of sorghum, the breeder is always
faced with the low genetic variability existing in the germplasm. To overcome
this constraint, a breeder may employ a breeding method like hybridisation and
selection between and within populations and/or use mutation breeding to provide
the desired variability. These procedures help to broaden the genetic base of
the breeding materials (Musoke et al., 1999). Parra-Negrette et al.
(1984) have evaluated induced mutation and hybridisation methods for producing
genetic variability in 15 quantitative characters of sorghum. Their results
showed large variability for plant maturity, plant height, panicle length and
exertion.
Much of the sorghum produced in Nigeria comes from the four
ecological zones of the country: Sahel, Sudan, Northern Guinea and Southern
Guinea savannas, where the rainfall is erratic and unpredictable and periods
of droughts frequent. This situation calls for the development of early maturing,
shorter and higher yielding sorghums that are resistant to disease and insect
pests. The objectives of this study, therefore, were to (i) study the genetic
variation in some agronomic characters of sorghum including the yield components,
(ii) study the relationship of these characters to one another, and (iii) study
the extent to which these characters are inherited.
MATERIAL AND METHODS
Genetic variability was created in sorghum "Short Kaura"
with gamma-ray from cobalt source (Co60). This variety is a medium plant of
about 1.5 m with broad leaf, thick stem, compact head (panicle) and large yellow
grains, and matures in about 125 to 150 days. Using genetic effective cell number
(GECN) of 2, and a mutation rate of 10-3, a total of 2300 seeds were irradiated
with 250 Gy as outlined by Nwasike (1985, unpubli.). Compensating for 70 per
cent viability of seeds, 65 per cent survival, and 80 per cent fertility after
treatment, the total number of seeds used in M1 were calculated as;
|
23000 |
|
M1 = |
|
= 3159 seeds |
|
2 x 0.80 x 0.70 x 0.65 |
|
For plant density of 500 per M2 in M1 plus 10 per cent for
planting control, the seeds required was calculated as;
3159 |
|
315.9 |
|
|
+ |
|
= 6.318 + 0.6318 = 6.949 seeds/m-2 |
500 |
|
500 |
|
Since 7 seeds/m-2 was the seeding rate and 20 seeds per row
in M2, the plot size was determined as 3159/7 seeds/m2 = 451.28 seeds m-2.
Treated M0 seeds were planted in the field during the off
season 1985. Selected M1 seeds were grown as bulk and, subsequently, bulked
seeds were grown as M2 population. Individual panicles or plants selected in M3 were planted ear-to-row for family selections. Ninety family lines were selected
in M3, after which 34 family selections were made in M4.
Thirty-four M4 family selections were threshed as individuals
parnicles and each divided into three equal portions to allow for the same materials
to be evaluated for three years. Each of the 3 portions served as a treatment,
and were evaluated in a randomised complete block design each year for three
years (1989-1991) at three sites of the Institute for Agricultural Research
farms, Ahmadu Bello University, Zaria, Nigeria. At each site there were four
replications with four row plots (6m long) and the plants were spaced 25 cm
within the row. Prior to ridging 32 kg N ha-1 of single superphosphate fertiliser
(P2O5) was broadcasted as basal dressing. Split application of nitrogen fertiliser
(Urea) was done, the first 32 kg N ha-1 was basal, while 32 kg N ha-1 was applied
3 weeks after thinning (top dressing).
Correlation coefficients between characters x1 and x2 were
calculated using the formula of Singh and Chaudhary (1977) as;
r. (x1x2) = |
|
_cov x1x2_ |
|
√ |
v (x1) .v(x2) |
where:
r (x1x2) = the correlation between characters x1 and x2
Cov (x1 x2) = the covariance between characters x1 and x2
V (x1) = the variance of character x1 and
V (x2) = the variance of character x2
Heritability. Estimates of broad sense heritabilities
were made from family means using variance components as given by the formula:
σ2g = genotypic
variance of a character
σ2ph = phenotypic variance
of a character
h2 = heritability of a character (broad sence)
RESULTS
The mean square values for the 22 characters for the individual
years as well as for the three years combined indicated that in 1989, all the
characters had highly significant (P=0.01) mean squares except protein percentage
and width of spikelet. A similar trend was observed for the mean squares of
the characters in 1990 and 1991 where we had highly significant (P=0.01) mean
squares for all the characters except for protein percentage and width of spikelet
which were not significant in the two years. There were also highly significant
(P=0.01) differences between years for all characters except width of spikelet.
Likewise, there were highly significant genotype x year interaction mean squares
for all characters except width of spikelet which showed a non-significant interaction.
Broad sense heritability estimates were computed for all characters
since they had positive σ2g,
basing on the components of variance estimates. Heritability from the combined
analysis (Table 1) showed that percentage protein which had almost the lowest
variance components for σ2g,
σ2ph, and σ2e
had the highest heritability value of 77.9%, while length of first leaf blade
had the lowest heritability value of 5.5% (Table 3).
Table 1.The genotypic (σ2g),
phenotypic (σ2ph), error variance
(σ2e) and heritability (h2)
estimates of 22 characters of sorghum plant types grown in three years (1989,
1990 and 1991) combined |
Character |
σ2g |
σ2ph |
σ2e |
σ2gy |
h2 (%) |
Protein percent (%) |
1.76 |
2.26 |
0.50 |
1.06 |
77.88 |
Grain weight/plant |
124.14 |
652.39 |
528.30 |
54.391 |
19.03 |
Length of panicle |
8.68 |
18.68 |
10.00 |
2.40 |
46.31 |
Spikelet number/panicle |
195.33 |
725.28 |
529.90 |
47.48 |
26.69 |
Grain weight/spikelet |
0.058 |
0.49 |
0.40 |
0.05 |
11.84 |
Days of 50% flowering |
6.49 |
13.60 |
7.10 |
6.49 |
47.72 |
Length of spikelet |
2.48 |
4.57 |
2.10 |
0.28 |
54.27 |
Width of spikelet |
0.0004 |
0.003 |
0.003 |
0.003 |
11.76 |
Length of first leaf blade |
3.85 |
69.56 |
65.70 |
4.44 |
5.50 |
Length of second leaf blade |
23.87 |
89.57 |
65.70 |
9.84 |
26.64 |
Length of third leaf blade |
23.45 |
59.80 |
36.4 |
17.61 |
39.21 |
Length of fourth leaf blade |
22.75 |
81.01 |
58.30 |
4.57 |
28.08 |
Length of first leaf sheath |
18.39 |
39.39 |
20.50 |
4.23 |
47.91 |
Length of second leaf sheath |
15.93 |
31.97 |
16.10 |
4.49 |
49.82 |
Length of third leaf sheath |
5.72 |
17.83 |
12.10 |
3.15 |
32.08 |
Length of fourth leaf sheath |
4.54 |
18.56 |
14.00 |
2.31 |
24.46 |
Length of first leaf internode |
5.22 |
14.53 |
9.30 |
1.41 |
35.92 |
Length of second leaf internode |
7.49 |
13.12 |
5.60 |
1.45 |
57.08 |
Length of third leaf internode |
7.48 |
12.72 |
5.24 |
1.99 |
58.81 |
Length of fourth leaf internode |
6.57 |
13.25 |
6.60 |
2.67 |
49.96 |
Length of fifth leaf internode |
8.45 |
13.65 |
5.20 |
2.73 |
61.90 |
Leaf angle in degrees |
11.54 |
154.33 |
126.70 |
-4.27 |
7.47 |
In the combined correlation matrix (Table 2) grain weight per
panicle was positively correlated to length of panicle, spikelet number per
panicle, grain weight per spikelet, days to 50% flowering, length of spikelet,
first and second leaf blades. Days to 50% flowering was negatively correlated
with all non-reproductive structures except length of 1st, 2nd and 3rd leaf
blades, and length of spikelet. Grain weight per spikelet was positively correlated
to length of spikelet, width of spikelet, length of 2nd, 3rd and 4th leaf blades,
lengths of 2nd and 3rd internode. Significant (P=0.05) correlations were found
between lengths of 1st and 2nd leaf sheaths, between 1st and 2nd internodes,
between 2nd and 3rd internodes and between 3rd and 4th and 5th internode. Highly
significant (P=0.01) correlations were also found between lengths of 2nd and
3rd leaf sheaths and between 3rd and 4th leaf sheaths.
TAble 2. Phenotypic correlation coefficients between 22 characters
in sorghum plant types combined over three (3) years, 1989, 1990 and 1991 |
|
2 |
3 |
4 |
5 |
6 |
7 |
8 |
9 |
10 |
11 |
12 |
13 |
14 |
15 |
16 |
17 |
19 |
20 |
21 |
22 |
23 |
1 |
-0.056 |
0.065 |
-0.104 |
0.021 |
-0.023 |
0.197 |
-0.060 |
-0.110 |
0.027 |
-0.024 |
-0.004 |
-0.040 |
-0.056 |
-0.046 |
-0.037 |
0.062 |
0.008 |
0.040 |
-0.023 |
0.004 |
0.071 |
2 |
1.000 |
0.068 |
0.260 |
0.328 |
0.023 |
0.068 |
0.064 |
0.096 |
0.092 |
-0.001 |
-0.015 |
-0.076 |
-0.097 |
-0.088 |
-0.057 |
-0.28 |
-0.030 |
-0.023 |
-0.058 |
-0.065 |
-0.004 |
3 |
|
1.000 |
0.067 |
-0.092 |
-0.146 |
0.441 |
0.038 |
0.008 |
0.057 |
0.174 |
0.174 |
0.225 |
0.235 |
0.208 |
0.223 |
0.191 |
0.198 |
0.212 |
0.209 |
0.219 |
-0.208 |
4 |
|
|
1.000 |
-0.083 |
-0.042 |
-0.155 |
0.068 |
0.130 |
-0.002 |
-0.004 |
-0.035 |
-0.036 |
-0.015 |
-0.076 |
-0.101 |
-0.184 |
-0.200 |
-0.115 |
-0.158 |
-0.199 |
-0.202 |
5 |
|
|
|
1.000 |
0.183 |
0.155 |
0.064 |
-0.033 |
0.140 |
0.035 |
0.101 |
-0.161 |
-0.235 |
-0.228 |
-0.154 |
-0.052 |
0.001 |
0.018 |
-0.011 |
-0.005 |
0.298 |
6 |
|
|
|
|
1.000 |
0.068 |
-0.006 |
0.331 |
0.226 |
0.040 |
-0.161 |
-0.733** |
-0.474 |
-0.380 |
-0.127 |
-0.187 |
-0.140 |
-0.045 |
-0.146 |
-0.097 |
0.115 |
7 |
|
|
|
|
|
1.000 |
0.068 |
0.050 |
0.175 |
0.118 |
0.144 |
0.026 |
0.085 |
0.073 |
0.207 |
0.218 |
0.290 |
0.261 |
0.205 |
0.273 |
0.039 |
8 |
|
|
|
|
|
|
1.000 |
0.097 |
0.138 |
0.038 |
0.089 |
-0.029 |
-0.004 |
-0.022 |
-0.011 |
0.015 |
0.052 |
0.009 |
-0.052 |
-0.040 |
-0.031 |
9 |
|
|
|
|
|
|
|
1.000 |
0.566 |
0.279 |
-0.003 |
-0.344 |
-0.103 |
-0.025 |
0.105 |
-0.132 |
-0.112 |
-0.107 |
-0.092 |
-0.083 |
-0.260 |
10 |
|
|
|
|
|
|
|
|
1.000 |
0.501 |
0.334 |
-0.219 |
-0.083 |
0.020 |
0.107 |
0.017 |
0.064 |
0.065 |
0.033 |
0.056 |
-0.005 |
11 |
|
|
|
|
|
|
|
|
|
1.000 |
0.481 |
0.060 |
0.125 |
0.109 |
0.096 |
0.050 |
0.042 |
0.084 |
0.066 |
0.112 |
0.039 |
12 |
|
|
|
|
|
|
|
|
|
|
1.000 |
0.240 |
0.196 |
0.155 |
0.098 |
0.168 |
0.252 |
0.201 |
0.197 |
0.203 |
0.146 |
13 |
|
|
|
|
|
|
|
|
|
|
|
1.000 |
0.689* |
0.577 |
0.305 |
0.293 |
0.289 |
0.277 |
0.289 |
0.318 |
-0.135 |
14 |
|
|
|
|
|
|
|
|
|
|
|
|
1.000 |
0.784** |
0.568 |
0.252 |
0.258 |
0.330 |
0.367 |
0.363 |
-0.257 |
15 |
|
|
|
|
|
|
|
|
|
|
|
|
|
1.000 |
0.716** |
0.212 |
0.247 |
0.294 |
0.302 |
0.349 |
-0.215 |
16 |
|
|
|
|
|
|
|
|
|
|
|
|
|
|
1.000 |
0.153 |
0.206 |
0.266 |
0.303 |
0.336 |
-0.180 |
17 |
|
|
|
|
|
|
|
|
|
|
|
|
|
|
|
1.000 |
0.624* |
0.485 |
0.454 |
0.373 |
0.037 |
18 |
|
|
|
|
|
|
|
|
|
|
|
|
|
|
|
|
1.000 |
0.673* |
0.581 |
0.536 |
0.079 |
19 |
|
|
|
|
|
|
|
|
|
|
|
|
|
|
|
|
|
1.000 |
0.652* |
0.628* |
0.074 |
20 |
|
|
|
|
|
|
|
|
|
|
|
|
|
|
|
|
|
|
1.000 |
0.634* |
0.023 |
21 |
|
|
|
|
|
|
|
|
|
|
|
|
|
|
|
|
|
|
|
1.000 |
0.041 |
22 |
|
|
|
|
|
|
|
|
|
|
|
|
|
|
|
|
|
|
|
|
1.000 |
*, ** Significant at 5% and 1% propability, respectively
|
I
|
II
|
III
|
1. Protein percent
|
9 Length of first leaf blade
|
17 Length of first internode
|
2 Grain weight per plant
|
10 Length of second leaf blade
|
18 Length of second internode
|
3 Length of panicle
|
11 Length of third leaf blade
|
19 Length of third internode
|
4 Spikelet number per panicle
|
12 Length of fourth leaf blade
|
20 Length of fourth internode
|
5 Grain weight per spikelet
|
13 Length of first leaf sheath
|
21 Length of fifth internode
|
6 Days to 50% flowering
|
14 Length of second leaf sheath
|
22 Leaf angle in degrees.
|
7 Length of spikelet
|
15 Length of third leaf sheath
|
|
8 Width of spikelet
|
16 Length of fourth leaf sheath
|
|
|
The results of principal component analysis for the different
characters are shown in Table 3. Seven characters accounted for 68% of the total
variance. These characters are length of second leaf sheath, length of third
leaf sheath, length of fourth leaf sheath, length of first internode, length
of second internode, length of third internode, length of fourth internode and
length of fifth internode. This indicates that these characters were the ones mostly affected by the irradiation, thus creating most of the variability in
them. Since these characters are positively correlated to most yield components,
they could be selected for as an indirect way of improving yield in our plant
types.
Table 3. Principal components (F) for the combined three (3)
years data |
Test |
F1 |
F2 |
F3 |
F4 |
F5 |
F6 |
F7 |
C2 |
1 |
0.017 |
0.087 |
0.195 |
0.020 |
-0.011 |
0.688 |
-0.029 |
0.521 |
2 |
-0.112 |
0.191 |
0.096 |
0.315 |
0.644 |
-0.216 |
0.332 |
0.729 |
3 |
0.142 |
0.121 |
0.219 |
0.046 |
0.423 |
0.451 |
-0.192 |
0.654 |
4 |
-0.194 |
-0.152 |
0.356 |
0.175 |
0.488 |
-0.351 |
-0.241 |
0.578 |
5 |
-0.161 |
0.434 |
-0.247 |
0.380 |
0.184 |
-0.083 |
0.520 |
0.730 |
6 |
-0.453 |
0.551 |
-0.022 |
-0.464 |
-0.031 |
0.051 |
0.125 |
0.742 |
7 |
0.333 |
0.442 |
-0.029 |
-0.046 |
0.309 |
-0.537 |
0.108 |
0.705 |
8 |
-0.005 |
0.157 |
0.162 |
0.183 |
0.200 |
-0.123 |
-0.200 |
0.179 |
9 |
-0.176 |
0.429 |
0.639 |
-0.322 |
-0.000 |
-0.133 |
-0.034 |
0.750 |
10 |
0.022 |
0.669 |
0.500 |
0.052 |
-0.188 |
-0.026 |
-0.020 |
0.738 |
11 |
0.185 |
0.449 |
0.463 |
0.338 |
-0.345 |
+0.043 |
-0.127 |
0.703 |
12 |
0.364 |
0.347 |
0.181 |
0.565 |
-0.293 |
-0.004 |
-0.154 |
0.714 |
13 |
0.691 |
-0.472 |
0.041 |
0.361 |
-0.019 |
+0.002 |
-0.008 |
0.833 |
14 |
0.748 |
-0.363 |
0.299 |
0.040 |
0.047 |
-0.022 |
0.168 |
0.813 |
15 |
0.710 |
-0.300 |
0.360 |
-0.075 |
-0.112 |
-0.003 |
0.323 |
0.846 |
16 |
0.589 |
-0.078 |
0.374 |
-0.277 |
-0.057 |
+0.064 |
0.445 |
0.775 |
17 |
0.594 |
0.181 |
-0.292 |
-0.040 |
0.082 |
-0.069 |
-0.289 |
0.791 |
18 |
0.685 |
0.303 |
-0.323 |
-0.082 |
0.088 |
-0.170 |
-0.204 |
0.736 |
19 |
0.700 |
0.307 |
-0.270 |
-0.158 |
0.103 |
-0.200 |
-0.071 |
0.681 |
20 |
0.705 |
0.216 |
-0.231 |
-0.179 |
0.043 |
-0.220 |
-0.039 |
0.639 |
21 |
0.704 |
0.240 |
-0.186 |
-0.176 |
-0.002 |
-0.125 |
0.067 |
0.639 |
22 |
-0.101 |
0.295 |
-0.526 |
0.333 |
-0.312 |
-0.037 |
0.235 |
0.620 |
Eigen value |
4.963 |
2.608 |
2.204 |
1.488 |
1.384 |
1.264 |
1.105 |
=15.0154 |
Total var. |
22.560 |
11.850 |
10.020 |
6.760 |
6.290 |
5.740 |
|
|
Common var |
33.05 |
17.37 |
14.67 |
9.90 |
9.22 |
8.22 |
7.36 |
=100.00 |
F = Principal components (PC) or Factors (F); C = Communality |
DISCUSSION
A genetic plant type may be defined as a genetically conditioned
association of morphologic traits (Morishima et al., 1967), represented
by a certain combination of leaf, stem (culm) and panicle characters. Growth
type or shape is easily recognised, but difficult to measure.
The result of the analysis of variance for the three years
combined showed significant (P=0.01) mean squares for years for all the characters
except for grain weight per spikelet and width of spikelet, an indication that
the yearly changes were high and could influence the performance of the genotypes
(Taye Kufa et al., 2001, this volume). The variances due to genotype
x year interactions were large, similar to the findings of Morishima and Oka
(1968), and indicate that the plants response to the growing seasons was genetically
controlled. According to Morishima et al. (1967), selection for seasonal
adaptability and for high yielding capacity may be made simultaneously within
a season. In cowpea, Ndiaga Cisse (2001, this volume) suggests that more than
one season is needed to identify genotypes adapted to semi-arid regions.
The genotypic variance was high for all the characters except
for percentage protein and width of spikelet. All other characters had lower
genotypic variances (σ2g) than their respective
phenotypic variances and error variances, except for percentage protein. This
probaly indicates that the gene controlling these characters are almost stable
at M4 generation, which tends to agree with the findings of Scossiroli
et al. (1960), Swaminathan (1961), Scossiroli and Polligrini-Scossiroli
(1962), Gaul (1964) and Borojevic (1963, 1965) who reported that variability
of quantitative characters in sorghum treated with mutagen increased up to four
times in the M2 and M3, gradually decreased in later generations
and stabilised around M5. Menkir et al. (1997) using RAPD
assessment method in some sorghum landrances found high genetic diversity within
the races bicolor and guinea, but low in the Kafor. All the characters showed
lower genotype x year interaction than their respective genotypic and phenotypic
variances. This suggests that the years were adequate for measuring the genetic
diversity of the characters (Quendeba et al., 1995). It also indicated
that the years did not influence the performances of the traits which make it
difficult to select for these traits in these particular years (Opeke and Fakorede,
1986).
Heritability estimates computed from the variance components
showed that percentage protein, which had almost the lowest variance components
had the highest heritability of 77.4 percent, in the combined years. This indicates
that their genotypic variances were higher or larger than their respective phenotypic
variances. This could mean that their genetic variation is large due to major
genes controlling the characters and that selection would be effective in the
desired direction for each of these traits in the population (Opeke and Fakorode,
1986).
A correlation matrix represents the inter-relationship of concerned
variates distributed in a multidimensional space (Morishima and Oka, 1968).
Length of panicle was positively correlated to all characters in the combined
correlation matrix except grain weight/spikelet and days to 50% flowering. Selecting
for the non reproductive structures which account for a large proportion of
the variations in the principal component analysis will likely improve yield
through increase in length of panicle and lengths of spikelet.
Quendeba et al. (1995) reported that grain yield and
spike yield of millet landraces were significantly correlated with spike length,
spike girth, stem diameter, spike number/plant, plant height and 1000 grain
weight. They also reported a negative correlation between spike length with
peduncle exertion, but positive correlation to spike yield and grain yield.
Principal component analysis indicated that seven factors accounted
for 68% of the total variation (Table 3). The first factor accounted for 22.6%
of the total variation and was also associated with length of 1st leaf sheath,
length of 2nd leaf sheath, length of 3rd leaf sheath, and 1st - 5th internodes
lengths. Factor 2 accounted for 11.85% of the total variation which is explained
by 1st leaf blade, 2nd leaf blade, 3rd leaf blade, days to 50% flowering, length
of spikelet and grain weight per spikelet. Thus, selecting for plants with long
leaf blades, which are tall and early maturing may lead to increase in grain
weight/spikelet (yield), as an indirect approach of improving yield in these
plant types.
REFERENCES
- Borojevic, K. 1963. Genetic advances in the height of induced micro and
macro mutations in the breeding of polyploid plants. In: Proceedings of
Symposium Application of Nuclear Energy in Agriculture, Rome, 1961: 241
pp.
- Borojevic, K. 1965. The effect of irradiation on the number of kernels per
spike in wheat, the use of induced mutations in plant breeding. Rep. FAO/IAEA.
Tech. Meeting Rome 1964), Pergaman Press, Oxford (1965) 504.
- FAO/ICRISAT, 1996. A world sorghum and millet economics: Facts, trends and
outlook. Food and Agricultural Organisation of the United Nations, Rome, Italy
and International Crops Research Institute for the Semi-Arid Tropics, Patancheru
502324, A.P. India. 68pp.
- Gaul, H. 1964. Mutation in plant breeding. Radiation Botany 4:155.
- Menkir, A. Peter, G. and Gebisa, E. 1997. RAPD based assessment of genetic
diversity in cultivated races of sorghum. Crop Science 37:564-569.
- Morishima, H., Oka, H.I. and Chung, T. 1967. Analysis of seasonal change
in genetic plant type. Japan Journal of Breeding.
- Morishima, H. and Oka, H. J. 1968. Analysis of genetic variation in plant
types of rice. III. Variations in general size and allometric pattern among
mutant lines. Japan Journal of Breeding 18:181-189.
- Musoke, C., Rubaihayo, P.R. and Magambo, M. 1999. Gamma rays and ethylmethane
sulphonate in vitro induced fusarium wilt resistant mutants in bananas.
African Crop Science Journal 7:313-320.
- NAERLS, 1996. Prospects and problems of the 1996 cropping season. A report
of a study conducted by the National Agricultural Extension and Research Liaison
Services (NAERLS) and Agricultural Planning, Monitoring and Evaluation Unit
(APMEU) 2 - 4 October, 1996. NAERLS, Ahmadu Bello University, Samaru, Zaria,
Nigeria. 62 pp.
- Ndiaga Cisse, 2001. Genotype x row spacing and environment interaction of
cowpea in semi-arid zones. African Crop Science Journal 9:359-367.
- Opeke, B.O. and Fakorede, M.A.B. 1986. Genetic variability, heritability
estimates, correlations and predicted response to S1 selection for seedling
emergence and yield in three maize populations. Nigerian Journal of Agronomy
1:1 - 4.
- Oke, O.L. 1976. The potential of millet and sorghum as food in Nigeria.
In: Proceedings of a Symposium on Sorghum and Millet for human food.
Vienna 11-12 November, 1976. IAEA, Vienna.
- Parra-Negrette, L.E., Mendoza, O. and Orfizcereceres, J. 1984. Comparison
of mutant lines. Parental collections and elite lines. North American Sorghum
Newsletter 29:14-15.
- Pickett, R.C. and Oswalt, D.L. 1971. Sorghum nutritional quality improvement:
In: Sorghum in Seventies. Oxford & IBH Publishing Co., New Delhi,
Bombay, Calcuta. pp. 445-463.
- Quendeba, B., Ejeta, G., Hanna, W.W. and Anand, K.K. 1995. Diversity among
African Pearl millet landrace populations. Crop Science 35:919 - 924.
- Scossiroli, R.E., Palenzona, O. and Rusmani, B. 1960. Radiation experiments
on Triticum durum and T. vulgare: Effects of ionizing radiations
on seeds. In: Proceedings of a Conference held at Karlscrune, IAEA, Vienna.
pp. 373-386.
- Scossiroli, R.E. and Polligrini-Scossiroli, S. 1962. Use of irradiation
applied to seed to induce new genetic variability for quantitative traits
in durum wheat. In: Symposium Genetic and Wheat Breeding. Agricultural
Research Institute, Hung Academy of Science. pp. 231-236.
- Singh, R.K. and Chaudhary, B.D. 1977. Biometrical Methods in Quantitative
Genetics Analysis. Kalyani Publishers, New Delhi - Ludiana. 317 pp.
- Swaminathan, M.S. 1961. Evaluation of the use of induced micro-and-macro
mutations in the breeding of polyploid crop plants. In: Symposium Application
of Nuclear Energy in Agriculture, Rome, 1961. IAEA, Vienna, Austria. pp.
241-277.
- Taye Kufa, Tesfaye Shimber, Alemsged Yilma, Anteneh Netsere and Endale Taye,
2001. The impact of close spacing on yield of arabica coffee under contrasting
agro-ecologies of Ethiopia. African Crop Science Journal 9:401-409.
|