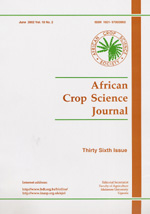
|
African Crop Science Journal
African Crop Science Society
ISSN: 1021-9730 EISSN: 2072-6589
Vol. 9, Num. 2, 2001, pp. 441-450
|
African Crop Science Journal, Vol. 9. No. 2, pp. 441-450
African Crop Science Journal, Vol. 9. No. 2, pp. 441-450
RESISTANCE OF MAIZE TO THE MAIZE WEEVIL: II. non-preference
J. Derera, P. Denash Giga1 and K.V. Pixley2 Department of Research & Specialist Services, P. O. Box CY550, Causeway, Harare, Zimbabwe 1University of Zimbabwe, P. O. Box MP 167, Mt. Pleasant, Harare, Zimbabwe 2CIMMYT-Zimbabwe, P. O. Box MP 163, Mt. Pleasant, Harare, Zimbabwe
(Received 9 June, 2000; accepted 14 December, 2000)
Code Number: CS01026
INTRODUCTION
The maize weevil (Stophilus zeamais Motsch.) is the
most destructive pest of stored maize in southern Africa. A survey of literature
indicates that there is much variation in maize for resistance to the maize
weevil. Exceptional grain resistance to maize weevil has been reported in indigenous
landraces from Mexico, Belize and some CIMMYT genotypes (Arnasson et al.,
1994), while Giga and Mazarura (1991) found significant variation among genotypes
from Malawi and Zimbabwe. Despite this, no significant attempts have been made
towards developing maize germplasm with improved resistance to storage pests.
The high levels of damage caused by insects in small farmer storage makes it
imperative that breeders identify sources of resistance and select for traits
that confer resistance (Smale et al., 1993).
Three resistance components of plants: antibiosis, non-preference
and tolerance to insect pests were reported by Painter (1951) and have been
studied and found to be important bases of grain resistance to maize weevil
(Gomez et al., 1982, 1983; Horber, 1989; Arnasson et al., 1997).
Non-preference is the heritable feature of the grain, which discourages insects
from feeding, colonising and oviposition or a combination of the three. Tipping
et al. (1986, 1987) reported large differences in attractiveness of maize
grain to attack by the maize weevil. Grain texture has been suggested (Tipping
et al., 1988) as the basis of non-preference resistance, because the
smooth pericarp may deter weevils from feeding and oviposition. Thus, these
studies suggest that the improvement of grain resistance to weevil should focus
mainly on antibiosis and non-preference mechanisms.
Small-scale farmers grow different maize varieties to reduce
the risk of grain losses due to several factors including drought and damage
by storage pests such as the maize weevil. Schulten (1975) reported that farmers
in Malawi grew both local varieties and commercial hybrids and that local varieties
were resistant, while improved varieties and hybrids were very prone to attack
by the maize weevil. Dobie (1974) also reported that the maize weevil, which
is a strong flier, is capable of exercising choice of grain among hybrids. Whereas
Kang et al. (1995) reported that the maize weevil preferred some crosses
for feeding, there is still limited genetic information on the basis of non-preference
resistance in maize grain. Knowledge of inheritance of non-preference resistance
to weevil feeding and oviposition would be helpful in designing breeding strategies
to develop resistant maize. Previous studies have been mainly confined to studying
grain resistance in no-choice tests in the laboratory, yet in the field insects
would select grain to feed on and for oviposition.
The objectives of this study were to evaluate non-preference
resistance to weevil in free-choice trials using F2 grain of experimental maize
hybrids and to study the gene action determining inheritance of this trait.
A companion paper (Derera et al., 2001, this volume) report results of
no-choice experiments studying inheritance of antibiosis type of weevil resistance
for the same hybrids.
MATERIALS AND METHODS
Formation of hybrids. Fifty-four experimental hybrids
from 18 inbred lines, six each from southern Africa, CIMMYT-Mexico and CIMMYT-Zimbabwe
(Table 1) were formed. The six inbred lines from CIMMYT-Mexico were derived
from four populations, which are adapted to lowland tropics, but could be grown
in Harare, Zimbabwe. These were previously screened and classified as resistant
to maize weevil at CIMMYT-Mexico, and therefore selected for our study. The
southern African lines were selected because of their adaptation in the region
and importance in regional breeding programmes. These lines have not previously
been screened for resistance to the maize weevil. The CIMMYT-Zimbabwe lines,
which were derived from populations with good yield and agronomic characteristics,
were selected because of their tolerance to maize streak virus disease, an economically
important disease in southern Africa.
TABLE 1. Pedigree and origin of inbred lines, and groups and
sub-groups of the lines used to form hybrids in a North Carolina Design
II mating |
Group |
Sub-group |
Inbred No. |
Pedigree |
Origin |
Mexico (Resistant) |
1 |
1 |
Rattray Arnold 8149 1-6-2-1-1-b-B |
CIMMYT-Mexico |
2 |
Rattray Arnold 8149 1-7-1-1-1-b-B |
CIMMYT-Mexico |
3 |
Pool 23QPM 5-9-5-2-1-b-B |
CIMMYT-Mexico |
2 |
4 |
Poza Rica 8121 7-12-1-1-1-b-B |
CIMMYT-Mexico |
5 |
Poza Rica 8121 7-2-5-1-1-b-B |
CIMMYT-Mexico |
6 |
Muneng 8128 14-11-2-1-1-b-B |
CIMMYT-Mexico |
Southern Africa (Regional) |
3 |
7 |
M162W-X |
South Africa |
8 |
SC Malawi (95A)-x-B |
Malawi/Zimbabwe |
9 |
NAW5867-X |
Zimbabwe |
4 |
10 |
M37W-X-X-6-X |
South Africa |
11 |
N3 Malawi (95A)-X-B |
Malawi/Zimbabwe |
12 |
I137TN-X-X-1-X |
South Africa |
CIMMYT-Zimbabwe (CIMMYT) |
5 |
13 |
CML202 |
CIMMYT-Zimbabwe |
14 |
CML216 |
CIMMYT-Zimbabwe |
15 |
FR810/TZMSRW-5-2-1-X-1-B |
CIMMYT-Zimbabwe |
6 |
16 |
CML206 |
CIMMYT-Zimbabwe |
17 |
[M37W/ZM6073#bF37sr-2-3sr-6-2X]-8-2-X-1-B |
CIMMYT-Zimbabwe |
18 |
[MSRXPOOL9]C1F2-176-4-1-4-X-X-B |
CIMMYT-Zimbabwe |
The lines were grouped according to their origin as Mexico
(Resistant; CIMMYT-Mexico), Regional (southern Africa) and CIMMYT (CIMMYT-Zimbabwe).
Further, the lines in each group were split into two sub-groups of three each,
using previous information on their pedigrees to allocate lines of the same
heterotic orientation into each sub-group. Inbred lines were numbered 1 to 18,
for convenience (Table 1), and hereafter, the lines and hybrids formed among
them are referred to and identified by these numbers plus abbreviated pedigrees.
Lines were crossed among sub-groups of three each according
to a North Carolina design II mating scheme (Comstock and Robinson, 1952), to
form nine crosses in each of the six sets, during winter of 1996 at Muzarabani.
Each inbred line was used as a male in one set (for three crosses) and as a
female for another set of crosses (for three different crosses), thus resulting
in a balanced mating design. The crossing of lines among sub-groups with different
heterotic orientation was conducted to maximise the likelihood of obtaining
high-yielding hybrids and to minimise the chance that any of the hybrids might
lack vigour (inbreeding). The 54 F1 experimental hybrids, plus local commercial
hybrids were full-sib mated to advance the seed to F2 grain, during summer (1996/97),
at CIMMYT station near Harare. We failed to obtain adequate F2 grain for two
hybrids (8x10 and 9x12), hence grain of 52 experimental hybrids was available
for the resistance screening.
Resistance screening (Free-choice test). The F2 grain
was used to evaluate non-preference resistance because this represents the generation
that is stored by farmers and will be vulnerable to attack by the weevil. In
addition to the 52 experimental hybrids, we included F2 grain of five local
commercial hybrids as reference entries. The commercial hybrid SR52 and a
Mexican composite Oaxaca 179 were used as the susceptible and resistant checks,
respectively. The maize weevils were obtained from CIMMYT-Zimbabwes laboratory
culture; these were F1 progeny of a field population collected from the University
of Zimbabwe farm, near Harare.
Two hundred grams of undamaged grain of each hybrid were kept
for 14 days in a freezer at 20°C to disinfest the grain of any prior field
infestations (Horber, 1989). Maize samples were then conditioned for six weeks
in a controlled temperature and humidity (CTH) room, which was maintained in
darkness at 28±2°C and 70±5% relative humidity.
Using a standard hole-puncher (for preparing paper for insertion
in a ring-binder notebook), we made four 3cm-diameter holes through both sides
of the small paper envelopes for the experiment. Fifty grams of conditioned
grain were placed inside, and the envelopes were then sealed, except for the
holes, which would allow weevil entry and exit. These envelopes were randomly
placed in an ovoid plastic container full of grain of a very weevil-susceptible
commercial hybrid. This grain had previously been infested with 500 weevils.
The envelopes were adequately spaced from each other to allow free movement
of weevils, and were held in approximately vertical (standing) position by the
grain of the susceptible hybrid filling the bottom of the container. This set-up
was replicated four times, with each replicate forming a randomised complete
block including the entire test hybrids and checks. Weevil-infested grain in
each replication (large plastic container) provided the source of insects for
the free-choice test. Grain samples had an equal chance of being infested, because
insects were randomly distributed in the grain bulk and all envelopes were spaced
within the container to allow ready access by insects. The containers were randomly
placed on the shelves in a CTH room and examined after 70 days.
This period allowed insects to feed and produce at least two
generations of progeny; the average development period of the maize weevil being
35 days, at optimal conditions (Gewinner et al., 1996). At the end of
the 70-day incubation period, the grain in each envelope was sieved, weighed
and the number of insects counted. The percentage grain weight loss in each
sample was calculated and Pearson phenotypic correlation coefficients between
grain loss, grain texture, and 100-seed weight were calculated.
For discussion purposes, the maize hybrids were classified
into five categories of resistance based on weight loss incurred by the susceptible
and resistant checks:
Resistant: |
grain weight loss < 2.0% (less than the resistant check).
|
Moderately resistant: |
grain weight loss between 2.1 and 4.0% (similar to the resistant
check). |
Moderately grain weight loss between susceptible: |
4.1 and 6.0% (less than susceptible check). |
Susceptible: |
grain weight loss between 6.1 and 8.0% (similar to susceptible
check). |
Highly susceptible: |
grain weight loss greater than 8.1% (greater than the susceptible
check). |
Statistical analyses. General analysis of variance (ANOVA)
for random effects model was conducted for grain weight loss data using the
SAS (1990) statistical package. The grain weight loss data were subjected to
the Design II analysis (Hallauer and Miranda, 1988), to estimate general (additive)
and specific combining ability (non-additive) effects for the hybrids within
and across sets. Variance of GCA effects was calculated for male (GCAm) and
female lines (GCAf). GCA effect for each line as male and female within its
respective sets of crosses, and SCA effect for each hybrid within its set of
crosses, were calculated as appropriate (i.e., when ANOVA indicated that variance
was statistically significant). Combining ability effects were fixed, while
replication effects were treated as random.
RESULTS AND DISCUSSION
Resistance screening. There were significant (P<0.01)
differences among hybrids for grain weight loss due to weevil damage during
the 70-day free choice exposure to weevils in the CTH room (Table 2). Weight
loss suffered by the experimental hybrids ranged from 0.85 to 8.45%. As expected,
the resistant check (Oaxaca 179) incurred significantly lower weight loss than
the susceptible check (SR52). The four commercial hybrids incurred weight losses
similar to SR52 (Table 2). When averaged for all hybrids within each set of
the Design II mating, weight loss ranged from 2.74% in the "resistant x
resistant" to 5.98% in the "regional x CIMMYT" set (Table 3).
TABLE 2. Grain weight loss of the 10 most resistant
and 10 most susceptible experimental hybrids from a Design II mating among
18 inbred maize lines, and for 6 reference entries |
Hybrid |
Abbreviated pedigree |
Hybrid set |
Grain weight loss (%) |
Rank |
Experimental hybrids |
6 x 2 |
Muneng8128 x RA8149 1-7 |
Res x Res# |
0.85 |
1 |
6 x 1 |
Muneng8128 x RA8149 1-6 |
Res x Res |
1.77 |
2 |
5 x 1 |
PR8121 7-2 x RA8149 1-6 |
Res x Res |
2.07 |
3 |
5 x 2 |
PR8121 7-2 x RA8149 1-7 |
Res x Res |
2.37 |
4 |
4 x 2 |
PR8121 7-12 x RA8149 1-7 |
Res x Res |
2.49 |
5 |
4 x 1 |
PR8121 7-12 x RA8149 1-6 |
Res x Res |
2.81 |
6 |
16 x 6 |
CML206 x Muneng8128 |
CIM x Res |
2.88 |
7 |
7 x 11 |
M162W x N3 |
Reg x Reg |
3.11 |
8 |
2 x 9 |
RA8149 1-7 x NAW5867 |
Res x Reg |
3.12 |
9 |
9 x 11 |
NAW5867 x N3 |
Reg x Reg |
3.33 |
10 |
18 x 6 |
MSRXPOOL9 x Muneng8128 |
CIM x Res |
6.32 |
44 |
13 x 18 |
CML202 x MSRXPOOL9 |
CIM x CIM |
6.43 |
45 |
12 x 15 |
I137TN x FR810/TZMSRW |
Reg x CIM |
6.79 |
49 |
3 x 8 |
Pool23QPM x SC |
Res x Reg |
6.95 |
51 |
18 x 5 |
MSRXPOOL9 x PR8121 7-2 |
CIM x Res |
7.20 |
52 |
11 x 14 |
N3 x CML216 |
Reg x CIM |
7.93 |
53 |
16 x 4 |
CML206 x PR8121 7-12 |
CIM x Res |
7.95 |
54 |
7 x 12 |
M162W x I137TN |
Reg x Reg |
7.95 |
55 |
10 x 14 |
M37W X CML216 |
Reg x CIM |
8.29 |
56 |
8 x 11 |
SC x N3 |
Reg x Reg |
8.45 |
57 |
Reference entries |
PAN695 |
|
|
6.72 |
48 |
CX5005 |
|
|
6.80 |
50 |
CX5003 |
|
|
6.02 |
41 |
SC501 |
|
|
6.43 |
46 |
Oaxaca 179 (Resistant) |
|
|
3.50 |
11 |
SR52 (Susceptible) |
|
|
6.62 |
47 |
Mean |
|
|
4.90 |
|
Significant (F) |
|
|
** |
|
LSD (5%) |
|
|
1.34 |
|
Refer to Table 1 for complete pedigree and origin of each
line
Note that ranks are not continuous because only the 10 most resistant
and the 10 most susceptible experimental hybrids and the 6 reference entries
are presented
# Res = resistant; CIM = CIMMYT-Zimbabwe; Reg = regional
** Significant at 1% probability level |
Table 3. Mean grain weight loss incurred by
hybrids within each set of a Design II mating among 18 inbred maize lines
|
Set |
Grain weight loss % |
Rank |
CIMMYT x CIMMYT |
4.69 |
3 |
CIMMYT x resistant |
5.24 |
4 |
Regional x CIMMYT |
5.98 |
6 |
Regional x regional |
5.63 |
5 |
Resistant x regional |
4.69 |
2 |
Resistant x resistant |
2.74 |
1 |
Mean |
4.82 |
|
LSD (0.05) |
0.47 |
|
The significant differences we recorded among hybrids for weevil
damage during this free choice experiment indicated that the maize weevil preferred
some hybrids relative to others. Small-scale farmers in Africa and Latin America
grow a combination of higher-yielding, improved maize varieties as well as a
wide range of traditional varieties. There is much anecdotal evidence that "local"
maize varieties, which are stored for planting from one season to the next,
bartered or shared among neighbours and handed-down from one generation to the
next of farmers, are generally less susceptible to weevil than "improved"
maize varieties. This might in fact be expected, because farmers inadvertently
(or deliberately) effect recurrent selection for weevil resistance, since each
season it is the undamaged grain that survives to germinate and produce the
next crop of grain and "seed". Giga and Mazarura (1991) have reported
preference of commercial hybrid grain by the maize weevil. Schulten (1975) and
Wright et al. (1989) have reported that farmers grow improved varieties
specifically for sale while the traditional maize, which is less prone to weevil
damage, is stored for home consumption. Although it was too small a sample to
justify conclusions about general weevil susceptibility of commercial or "improved"
varieties, the four commercial hybrids we evaluated were susceptible and similar
to the susceptible check for damage by weevil (Table 2).
Based on our classification, hybrids 6x1 and 6x2 were classified
as resistant, while 10x14 and 8x11 were highly susceptible to the maize weevil
(Table 2). Frequency distribution of the 52 experimental hybrids for weight
loss was approximately normal, with 4% resistant, 29% moderately resistant,
44% moderately susceptible, 19% susceptible and 4% highly susceptible hybrids.
This wide variation of genotypes for resistance to maize weevil indicated segregation
of resistance genes in the F2 generation hybrids. This result is similar to
Dobies (1977) categorisation of 217 genotypes, which showed normal distribution
with 20% of the genotypes at the lower end of the weevil susceptibility index
scale (resistant), 30% moderately resistant/susceptible, 30% susceptible and
20% highly susceptible.
Genetic basis of weevil resistance. Mean squares of
GCA for lines used as males (GCAm), lines used as females (GCAf) and SCA of
hybrids for grain weight loss across sets were highly significant (P<0.01)
(Table 4). GCA and SCA effects were of similar importance for explaining differences
of grain weight loss among hybrids, accounting for 47 and 53% of sum of squares
for hybrids, respectively. GCAm (25%) and GCAf (22%) effects across sets were
of similar magnitude.
TABLE 4. Mean squares from analysis of variance
across sets, and for each set of a Design II mating among 18 inbred maize
lines for grain weight loss (%) of hybrids caused by weevils during a non-preference
experiment |
Source of Variation |
Across sets |
Resx Reg |
CIM x Res |
Res x Res |
Reg x CIM |
Reg x Reg |
CIM x CIM |
df |
MS |
df |
Mean square |
Sets |
5 |
45.96** |
|
|
|
|
|
|
|
Replication |
18 |
17.68** |
3 |
11.90** |
12.40** |
8.83** |
38.30** |
17.30** |
17.40** |
F2 Hybrids |
46 |
7.69** |
8 |
5.91** |
10.80** |
5.58** |
7.70** |
15.30** |
2.79** |
GCA female |
12 |
6.21** |
2 |
13.80** |
3.99 |
1.63** |
4.03* |
11.70* |
2.10 |
GCAmale |
12 |
7.11** |
2 |
2.73** |
1.59 |
17.00* |
7.46** |
7.36 |
6.53** |
SCA |
22 |
8.05** |
4 |
3.55** |
18.70** |
1.85** |
9.66** |
18.40** |
1.26 |
Error |
138 |
1.03 |
24 |
0.44 |
1.51 |
0.30 |
1.11 |
2.34 |
0.82 |
Res = resistant; CIM = CIMMYT-Zimbabwe; Reg = regional
F2 Hybrid and error d.f = 6 and 18 respectively
*, ** Significant at 5% and 1% probability level, respectively |
The significant GCA for weight loss indicates that additive
gene action was important in determining non-preference resistance to maize
weevil. Equal importance of GCAm and GCAf indicated that maternal effects were
not important in determining non-preference. Thus both parents were contributing
similar levels of resistance to these hybrids. Furthermore, significant SCA
for grain weight loss among hybrids indicated that non-additive gene action
was important in determining non-preference of some hybrids. However, similar
male and female additive effects for non-preference were in contrast with Kang
et al.s (1995) findings in which maternal effects were significant for
non-preference of F2 hybrids by the weevil in free-choice tests.
Negative GCA for grain weight loss indicates non-preference,
while positive GCA indicates preference of hybrids of a given line by the weevil
(Table 5). Inbred lines 2 and 9 had significant negative GCA for weight loss
as both male and female, indicating that these inbred lines contributed more
than average levels of non-preference type of resistance to maize weevil as
both pollen sources and seed parents. It is important to remember that performance
of lines in this experiment is only relative to the other lines used within
the same set of hybrids; therefore, inferences about the broader value of lines
in breeding programmes should be made with caution.
TABLE 5. Estimates of general combining ability
effects (GCA) for grain weight loss (%) for lines with significant GCA as
females or males within sets of Design II mating among 18 inbred maize lines
|
Inbred No. |
Abbreviated pedigree |
Hybrid set |
GCA |
S.E(±) |
(%) |
Lines used as females |
2 |
RA8149 1-2 |
Res x Reg# |
-1.1** |
0.2 |
3 |
Poo123QPM |
Res x Reg |
1.1** |
0.2 |
6 |
Muneng 8128 |
Res x Res |
-0.4** |
0.1 |
8 |
SC |
Reg. X Reg |
1.7** |
0.4 |
9 |
NAW 5867 |
Reg. Reg |
-1.5** |
0.4 |
12 |
1137TN |
Reg x CIM |
-0.6* |
0.3 |
Lines used as males |
1 |
RA8149 1-6 |
Res x Res |
-0.5** |
0.1 |
2 |
RA8149 1-7 |
Res x Res |
-0.8** |
0.1 |
3 |
Poo123QPM |
Res x Res |
1.3** |
0.1 |
7 |
M162W |
Res x Reg. |
0.3* |
0.2 |
9 |
NAW5867 |
Res x Reg. |
-0.5** |
0.2 |
13 |
CML202 |
Reg. x CIM |
-0.7* |
0.3 |
14 |
CML216 |
Reg. x CIM |
0.9** |
0.3 |
17 |
M37W/ZM607 |
CIM x CIM |
0.5** |
0.2 |
18 |
MSRXPOOL9 |
CIM x CIM |
0.8** |
0.2 |
Refer to Table 1 for complete pedigree and origin of each
line
S.E = standard error
#Res = resistant; CIM = CIMMYT-Zimbabwe; Reg = regional.
*, ** Significant at 5% and 1% probability level, respectively |
Regional inbred lines (9 and 12) that had negative GCA for
non-preference resistance to weevil as male or female might be directly useful
in regional programmes because they are adapted to southern Africa. Moreover,
Mexican weevil-resistant lines that showed good GCA for non-preference resistance
could be used as sources in breeding projects to improve regionally adapted
and elite lines. Negative (favourable) SCA effects indicated that some hybrids
had unexpectedly good performance for non-preference by the maize weevil (Table
6). The significant negative SCA effects for weight loss imply that greatest
non-preference resistance to weevil will be obtained in hybrids that are combinations
of lines that exploit non-additive gene action, or heterosis for this trait.
Table 6. Estimates of specific combining ability
effects (SCA) for grain weight loss (%) for hybrids with significant SCA
within sets of a Design II mating among 18 inbred maize lines |
Hybrid |
Abbreviated Pedigree |
Hybrid set |
SCA (%) |
S.E.(±) |
1 x 8 |
RA8149 1 6 x SC |
Res x Reg# |
-1.5** |
0.2 |
1 x 9 |
RA8149 1-6 x NAW5867 |
Res x Reg. |
0.8* |
0.2 |
3 x 8 |
Poo123QPM x SC |
Res x Reg |
1.0* |
0.2 |
3 x 9 |
Poo123QPM x NAW5867 |
Res x Reg |
-0.9** |
0.2 |
4 x 1 |
PR8121 7-12 x RA8149 1-6 |
Res x Res |
0.4* |
0.2 |
4 x 2 |
PR8121 7 12 x RA8149 1-7 |
Res x Res |
0.4* |
0.2 |
4 x 2 |
PR8121 7-12 x P99123QPM |
Res x Res |
-0.7** |
0.2 |
6 x 2 |
Muneng8128 x RA8149 1-7 |
Res x Res |
-0.6** |
0.2 |
6 x 3 |
Muneng 8128 x Poo123QPM |
Res x Res |
0.4* |
0.2 |
8 x 11 |
SC x N3 |
Reg x Reg |
1.4* |
0.5 |
8 x 12 |
SC x 1137TN |
Reg x Reg |
02.3** |
0.5 |
9 x 10 |
NAW5867 x M37W |
Reg. x Reg |
1.4* |
0.5 |
10 x 14 |
M37W x CML216 |
Reg x CIM |
1.2** |
0.4 |
10 X 15 |
M37W x FR810/TZMSRW |
Reg x CIM |
-0.9* |
0.4 |
12 x 15 |
I137TN x R810/TZMSRW |
Reg x CIM |
1.6** |
0.4 |
16 x 4 |
CML206 x PR8121 7-12 |
CIM x Res |
2.7** |
0.4 |
16 x 5 |
CML206 x PR8121 7-2 |
CIM x Res |
-0.9* |
0.4 |
16 x 6 |
CML206 x Muneng8128 |
CIM x Res |
-1.8** |
0.4 |
18 x 4 |
MSRXPOOL9 x PR8121 7-12 |
CIM x Res |
-2.7** |
0.4 |
18 x 5 |
MSRXPOOL9 x PR8121 7-2 |
CIM x Res |
1.2* |
0.4 |
18 x 6 |
MSRXPOOL9 x Muneng 8128 |
CIM x Res |
0.9* |
0.4 |
Refer to Table 1 for complete pedigree and origin of each
line
S.E. = standard error
#Res = resistant; CIM=CIMMYT-Zimbabwe; Reg=regional
*, ** Significant at 5% and 1% probability level, respectively |
Our results concur with the findings of Kang et al.
(1995) that additive and non-additive gene action are important in conditioning
non-preference resistance of grain to maize weevil. Whereas our study did not
investigate the chemical basis for grains non-preference resistance, Schoonhoven
et al. (1976) suggested that non-preference was based on the lack of
feeding stimulants in resistant kernels. Furthermore, Gomez et al. (1982,
1983) reported that the inbred line A619 attracted more weevils for oviposition,
and contained higher ethanol content, which was suggested to be a weevil stimulant.
Our results suggest that it is possible to develop hybrids with improved non-preference
resistance of F2 grain to maize weevil. Practical considerations, such as the
substantial time and money costs of implementing a weevil resistance selection
scheme within an applied maize breeding programme, are likely to result in hesitation
among maize breeders to pursue this goal. We believe that more research is needed
to confirm these results using a broader spectrum of maize germplasm, and that
this research is most suitably conducted within the public sector, at institutions
such as ours (CIMMYT and the University of Zimbabwe).
ACKNOWLEDGEMENT
We gratefully acknowledge The Rockefeller Foundation for funding
this project.
REFERENCES
- Arnasson, J. T., Conilh de Beyssac, B., Philogene, B.J.R., Bergvinson, D.,
Serratos, J.A. and Mihm, J.A. 1997. Mechanisms of resistance in maize grain
to the maize weevil and the larger grain borer. In: Insect resistant maize:
Recent advances and utilisation: Proceedings of an International Symposium
held at the International Maize and Wheat Improvement Centre (CIMMYT)
27 November 3 December, 1994. Mihm, J.A. (Ed.), pp. 91-95. Mexico, D.F.:
CIMMYT.
- Arnasson, J. T., Baum, B., Gale, J., Lambert, J. D. H., Bergvinson, D.,
Philogene, B. J. R., Serratos, J. A., Mihm, J. and Jewel, D.C. 1994. Variation
in resistance of Mexican landraces of maize to maize weevil, Sitophilus
zeamais, in relation to taxonomic and biochemical parameters. Euphytica
74:227-236.
- Comstock, R. E. and Robinson, H.F. 1952. Estimation of average dominance
of genes. In: Heterosis. Gowen, J.W. (Ed.), pp. 494-516 . Iowa State
University Press Ames, Iowa, USA.
- Derera, J., Pixley, K.V. and Giga, D. P. 2001. Resistance of maize to the
maize weevil: 1. Antibiosis. African Crop Science Journal 9:431-440.
- Dobie, P. 1974. The laboratory assessment of the inherent susceptibility
of maize varieties to post harvest infestation by Sitophilus zeamais.
Journal of Stored Product Research 10:183-197.
- Dobie, P. 1977. The contribution of the Tropical Stored products Centre
to the study of insect resistance in stored maize. Tropical Stored Products
Information 34:7-21.
- Gewinner J., Harnisch, R. and Muck, O. 1996. Manual of the Prevention
of Post-Harvest grain losses. Post-Harvest Project. GTZ, Eschborn. 330
pp.
- Giga, D.P. and Mazarura, U.W. 1991. Levels of resistance to the maize weevil,
Sitophilus zeamais (Motsch.) in exotic, local open-pollinated and hybrid
maize germplasm. Insect Science and its Applications 12:159-169.
- Gomez, L. A., Rodriguez, J.G., Poneleit, C.G. and Blake, D.F. 1982. Preference
and utilisation of maize endosperm variants by the rice weevil. Journal
of Economic Entomology 75:363-367.
- Gomez, L. A., Rodriguez, J.G., Poneleit, C.G., Blake, D.F. and Smith, C.R.
Jr. 1983. Chemosensory responses of the rice weevil (Coleoptera: Curculionidae)
to a susceptible and a resistant corn genotype. Journal of Economic Entomology
76:1044-1048.
- Hallauer, A.R. and Miranda, J.B. 1988. Quantitative genetics in maize
breeding. 2nd Edition. Iowa State Universitiy Press, Ames, Iowa, USA.
- Horber, E. 1989. Methods to dect and evaluate resistance in maize to grain
insects in the field in storage. In: Towards insect resistance maize for
the Third World. Proceedings of the International Symposium on metho-dologies
for developing host plant resistance to maize insects. Mexico, D.F. CIMMYT.
327 pp.
- Kang, M.S., Zhang, Y. and Magari, R. 1995. Combining ability for maize weevil
preference of maize grain. Crop Science 35:1556-1559.
- Painter, R. H. 1951. Insect resistance in Crop Plants. McMillian,
New York. 520 pp.
- SAS Institute Inc., 1990. SAS Procedures Guide Version 6, Third Edition,
Cary, North Carolina, USA.
- Schoonhoven, A. V., Horber, E. and Mills, R.B. 1976. Conditions modifying
expression of resistance of maize kernels to the maize weevil. Environmental
Entomology 5:163-168.
- Schulten, G.C. 1975. Losses in stored maize in Malawi (C. Africa) and work
undertaken to prevent them. Eppo. Bulletin 5:113-120.
- Smale, M., Kaunda, Z.H.W., Makina, H.L. and Mkandawire, M.M.M.K. 1993.
Farmers evaluation of newly released maize cultivars in Malawi: A comparison
of local maize, semi-flint and dent hybrids. CIMMYT- Malawi & Zimbabwe.
- Tipping, P. W., Legg, D. E., Rodriguez, J. G. and Poneleit, C. G. 1988.
Influence of maize pericarp surface relief on resistance to the maize weevil
(Coleoptera: Curculionidae). Journal of Kansas Entomological Society
61: 237-241.
- Tipping, P. W., Mikolajczak, K. L., Rodriguez, J. G., Poneleit, C. G. and
Legg, D. E. 1987. Effects of whole corn kernels and extracts on behaviour
of maize weevil (Coleoptera: Curculionidae). Journal of Economic Entomology
80:1010-1013.
- Tipping, P. W., Mikolajczak, K. L., Rodriguez, J. G., Zilkowski, B. W. and
Legg, D. E. 1986. Attraction of Sitophilus oryzae (L.) and S. zeamais
Motsch. (Coleoptera: Curculionidae) to extracts from two corn genotypes. Journal
of the Kansas Entomological Society 59:190-194.
- Wright, V. F., Mills, R. B. and Willcutts, B. J. 1989. Methods for culturing
stored grain insects. In: Toward insect resistance maize for the Third
World. Proceedings of the International Symposium on methodologies for developing
host plant resistance to maize insects. Mexico, D.F. CIMMYT. 302 pp.
|