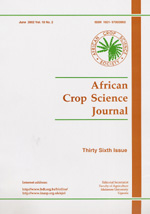
|
African Crop Science Journal
African Crop Science Society
ISSN: 1021-9730 EISSN: 2072-6589
Vol. 11, Num. 2, 2003, pp. 55-64
|
African Crop Science Journal, Vol. 11. No. 2, 2003, pp. 55-64
SELECTION FOR GRAIN YIELD IN SORGHUM UNDER
MOISTURE AND NUTRIENT STRESS ENVIRONMENTS
ABEBE MENKIR and GEBISA EJETA1
International Institute for Tropical Agriculture, Ibadan,
Nigeria
1Department of Agronomy, Purdue University, 1150 Lilly Hall of Life
Sciences, West Lafayette, IN 47907-1150
(Received 17 December, 2001; accepted, 20 December 2002)
Code Number: cs03008
ABSTRACT
Crop breeders in the semi-arid tropics often confront a combination of moisture
and nutrient stress in their target production environments. Stability
of performance under stress conditions is, thus, a desirable goal for crop
improvement. The objectives of these studies were (1) to explore
the extent of genetic variability among recombinant inbred lines of sorghum (Sorghum
bicolor) in high and low fertility as well as in irrigated and
rainfed conditions, and (2) to predict and measure the correlated responses
of sorghum yields when selection is exercised in either one environment or
a combination of contrasting environments. We evaluated 57 unselected
recombinant inbred (RI) sorghum lines. Large differences in yield,
height, and maturity were detected among lines in each contrasting environment. Genetic
variance and heritability estimates for each trait in each stress environment
did not differ significantly from those in the corresponding non-stress environments. For
improving yield under stress, indirect selection in high fertility or in the
irrigated environment was less efficient than direct selection in the corresponding
stress environment (low fertility or rainfed). When indirect selection
involved yield combinations from low and high fertility or rainfed and irrigated
conditions, at least five lines appeared among the 10 top-ranking
lines of each contrasting environment. Thus, greater gain in performance
over contrasting environments may be achieved by selecting for yield in more
than one environment, rather than by selecting in any single environment.
Key Words: Crop improvement, genetic variance, heritability, irrigated,
rainfed
RÉSUMÉ
Les reproductions de plante dans les tropiques semi-arides sont
souvent confrontés à la combinaison de l'humidité et au stress des substances
nutritives dans leurs environnements de productions visés. La stabilité de
performance sous des conditions de stress est, ainsi, un objetif désirable
pour l'amélioration de plante. Les objectifs de ces études étaient (1) d'explorer
l'étendue de la variabilité génetique entre les races consanguines des recombinants
de sorgho (Sorghum bicolor) en forte et faible fertilité ainsi que
dans des conditions d'irrigation et pluies, et (2) prédire et mesurer les réponses
corrélées des rendements de sorgho quand la sélection est exercée dans un seul
environnement ou une combinaison contrastée d'environnements. Nous avons évalué 57
lignes consanguines des recombinants de sorgho non sélectionnées (RI). Des
larges différences en rendement, hauteur et maturité étaient detectées entre
les races dans chaque environnement contrasté. La variance génetique et les
prévisions d'héritage pour chaque trait et chaque stress d'environnment n'ont
pas différé de manière significative des correspondants en environnments non
stressés. Pour améliorer le rendement sous stress, la sélection indirecte en
haute fertilité ou en environnemnt irrigué étaient moins efficace que les sélection
directe en environnement correspondant stressé (faible fertilité ou pluie).
Quand la sélection indirecte a impliqué des combinaisons de rendements de faible
et fertilité élevée ou conditions pluvieuses et irriguées, au moins cinq races
ont apparu pami les 10 premiers classements des races de chaque environnement
contrasté. Ainsi, un grand gain en performance au-délà des environnements contrastés
peut- être atteint par sélection pour rendement dans plus d'un environnement,
plutot que par sélection dans n'importe un seul environnement.
Mots Clés: Amélioration de la plante, variance génetique, en héritage,
irriué, pluvieux
INTRODUCTION
Sorghum (Sorghum bicolor (L.) Moench) is an important food crop in
the semi-arid tropics (SAT), where rainfall is generally insufficient
and erratic (Rasenberg, 1984), and soil fertility status is very poor (Lal,
1987). To improve and stabilise crop production in these areas,
the genetic potential of crop germplasm needs to be adjusted to
available environmental resources (Kawano and Jennings, 1983).
Strategies are available for improving performance in nutrient deficient
and moisture deficit soils of the SAT (Frey, 1964). One of the approaches
assumes that selection of plant genotypes under optimal nutrient and moisture
supply may maximise genetic gain in low input production environments. Testing
the usefulness of this approach will be important both in the stress-prone
SAT as well as in temperate environments where stress is infrequent but farmers
often look for ways to reduce production costs (Atlin and Frey,
1989).
A review by Bramel-Cox et al. (1991) presented conflicting results
regarding the usefulness of selection under non-stress conditions to identity
genotypes for use in low input environments. The amount of genetic
progress from selection for broad adaptation in both favourable and adverse
production conditions diminishes as the intensity and frequency of stress increases
in the unfavourable production environments (Bramel-Cox et al., 1991;
Zavala-Garcia et al., 1992). Others have found that initial
selection under limited nitrogen supply would not improve the probability of
identifying crop genotypes with wide adaptation to both high and low fertility
conditions (Seetharama et al., 1984). These conclusions were
drawn from sorghum studies which were conducted in either moisture stress (Bramel-Cox et
al., 1991; Zavala-Garcia et al., 1992) or limited soil fertility
(Seetharama et al., 1984). However, crop breeders in the
semi-arid tropics most frequently confront a combination of moisture and nutrient
stresses in their target production environments. Therefore, evaluating
breeding materials under both limited moisture and nutrient supply may increase
the chance for identifying lines which are adapted to one or both stress conditions.
The breeding procedure commonly practised for handling segregating generations
affects the rate of genetic progress that can be made under stress. Single
plants selected from early segregating generations in nutrient deficient and
moisture deficit soils may fail to maintain the same expression in subsequent
progeny testing because of the inherent lack of uniformity in the intensity
of these stresses in the experimental field during selection as well as because
of the resultant segregation from single plants. To minimise these
problems, evaluation and selection for stress tolerance may be delayed until
true breeding lines are developed. The use of the single seed descent
breeding method which allows rapid attainment of homozygosity may, therefore,
be suitable for developing recombinant inbred (RI) lines to be tested
under an array of contrasting moisture and nutrient environments. This was
the subject of investigation in this study.
Therefore, the objectives of this study were: (1) to explore the
extent of genetic variability among unselected single seed derived recombinant
inbred (RI) lines grown in high and low fertility as well as in irrigated and
rainfed conditions, and (2) to predict and measure correlated responses of
yield when selection is exercised in either one environment or a combination
of contrasting environments.
MATERIALS AND METHODS
Fifty-seven random RI lines of sorghum derived via single seed descent
from the F6 generation of a cross between two sorghum cultivars; namely,
K886 and CS3541, were used as experimental materials. Cultivar K886
was an inbred line tolerant to pre-flowering drought stress, while CS3541 was
chosen for susceptibility to pre-flowering drought stress and its good general
combining ability and production of high yield potential hybrids under stress-free
conditions. The responses of these two sorghum inbred lines to limited
nutrient supply was unknown.
The 57 RI lines, the two parents, and three checks with tolerance to either
pre- or post-flowering drought stress were grown at Lubbock under rainfed and
irrigated conditions in 1990 and 1991. When sorghum is grown under
rainfed conditions at Lubbock, it normally experiences pre-flowering drought
stress. In the irrigated experiment, supplementary irrigation was
applied from planting to flowering, whenever it was deemed necessary. The
same sets of lines were also planted at Lafayette under high and low fertility
treatments in 1990 and 1991. In each year, the low fertility experiment
was planted in the field which received no fertiliser for over 35 years. The
high fertility experiment received 150 kg N, 40 kg P, and 50 kg K per hectare.
Genotypes were arranged in a randomised completed block design and were replicated
four times in each test environment (rainfed, irrigated, low-and high-fertility). Because
of limited seed supply, however, only 44 and 54 inbred lines were common to
the two years at Lafayette and Lubbock, respectively. Each line
was planted in a single row of 3 to 5 m long spaced 76 cm apart. The
experiments were planted either in late May or in early June of each year.
All plots were harvested at maturity and grain yield was measured as the
weight of threshed grain from a plot expressed in kg ha-1. Days
to flowering were recorded as the number of days from planting to the date
when 50% of the plants in a plot were shedding pollen. Plant height was
measured as the distance from the soil surface to the tip of the panicles in
each plot.
Analyses of variance combined over years were computed for each contrasting
environment from standardised data. The raw data of each trait within
each environment and year was standardised, with a block mean of zero and standard
deviation of one (Fox and Rosielle, 1982). This was done to remove
the effects of large differences in scale on comparison of variances and predicted
gains from selection between stress and non-stress environments.
Inbred lines and years were considered to be random effects. Genetic
variances were estimated from mean squares among RI lines and their interaction
with year in the analysis of variance. Standard errors of the genetic
variances were calculated using the method of Anderson and Bancroft (1952).
The heritability of each contrasting environment was calculated on an entry-mean
basis as a ratio of genotypic variance to phenotypic variance for each trait.
The standard errors for heritabilities were computed according to the method
of Hallauer and Miranda (1988).
Genetic covariance between stress and non-stress environments for each trait
was calculated using the method of Atlin and Frey (1989). The
genetic correlation between two contrasting environments for yield, plant height,
and days to flowering was estimated by using the formula of Falconer (1989). Standard
errors for the genetic correlations were computed according to the method of
Mode and Robinson (1959). The magnitude of gain from selection in a non-stress
environment which was expected to be expressed in a stress environment was
predicted using the formula of Falconer (1989) given below:
CRs =iσsub>shshnsrg,
where:
CRs = correlated response in stress environments to
selection in non stress environments
i = selection intensity
σs = square root of
phenotypic variance under stress
rg = genetic correlation between stress
and non stress environment
hs = square root of heritability in stress environments
hns = square root of heritability in non stress
environments
Simple correlations among the three agronomic traits were calculated for
all test environments.
A rank summation index was used to optimise genetic gain in a single environment
or two contrasting environments. The index scores for the lines within each
contrasting environment were calculated as the sum of ranks of the line mean
yields in the two years. Index scores for a combination of two contrasting
environments were also computed as:
RSI = Rank (90 yield-stress) + Rank (91 yield-stress) + Rank (90 yield-non-stress)
+ Rank (91 yield-non-stress).
The actual response of indirect versus direct selection was measured
by comparing the mean performance of the top-ranking 10 lines which were selected
in a single environment or a combination of environments with the mean response
of the same set of lines selected in a single environment. The mean
of the selected lines in a single environment or a combination of environments
was expressed as a percentage of the overall mean of all the random lines which
were tested in each response environment. Because year to year variation is
inherently high in stress-prone production environments, a selection scheme
that takes advantage of genotype by year interaction may prove useful. A
rank summation index which assigned equal weights to the two years in each
environment as well as in a combination of contrasting environments was used
to evaluate the actual yield advances from indirect and direct selections.
RESULTS AND DISCUSSION
Yields of all RI lines, their parents, and checks in the low fertility environment
were significantly (P<0.05) smaller than those in high fertility
environment (Table 1). Low soil fertility reduced yields of all
genotypes to 46% of the high fertility environment. The overall
mean yield of all genotypes in the rainfed environment was reduced to 69% of
the irrigated environment. Grain yields under irrigation were unexpectedly
low because of the limited irrigation water supply. The recombinant
inbred lines, their parents, and checks did not differ significantly (P>0.05)
for grain yields in each contrasting environment (data not shown). Soil
fertility as a factor did not differ significantly (P>0.05) for plant heights
of the inbred lines, their parents, and checks. By contrast, plants
from each group grew significantly taller in the irrigated than in the rainfed
environment. The differences in mean days to flowering between high
and low fertility and between rainfed and irrigated environments were not significant
(Table 1).
TABLE 1. Mean grain yields, days to flowering, and plant height of single-seed
derived F6 lines, their parents, and inbred checks grown under high and low
fertility as well as rainfed and irrigated conditions*
Environment |
Recombinant inbred lines |
Parent |
Check |
|
Min |
Max |
Mean |
Mean |
Mean |
Grain yield (Mg ha-1) |
|
|
|
|
|
Low fertility | 1.44 | 4.84 | 2.84a** | 3.96a | 2.67a |
High fertility | 3.42 | 9.16 | 6.46b | 7.72b | 6.44b |
Rainfed | 1.08 | 2.94 | 1.94a | 2.34a | 1.96a |
Irrigated | 1.25 | 4.64 | 2.94b | 3.23b | 2.92b |
Plant height (cm) |
Low fertility | 68 | 184 | 124a | 106a | 109a |
High fertility | 65 | 194 | 126a | 108a | 113a |
Rainfed | 60 | 158 | 98a | 87a | 80a |
Irrigated | 64 | 192 | 123b | 108b | 102b |
Days to flowering |
Low fertility | 68 | 92 | 76a | 76a | 76a |
High fertility | 70 | 85 | 76a | 75a | 74a |
Rainfed | 58 | 69 | 64a | 63a | 62a |
Irrigated | 56 | 72 | 66b | 66a | 63a |
*Pooled data, obtained over a 2 year period.
**Means within a column followed by the same letter were not significantly different
at P=0.05 level using t-test
Mean yield of the highest yielding RI line was more than twice that of the
lowest yielding recombinant inbred line in each test environment (Table 1). In
spite of such large differences in grain yield, the genetic variance in high
fertility environment was not significant because of large year x line interaction
(Table 2). Since the 1991 cropping season was dry at Lafayette,
it could contribute to the large year x line interaction. The genetic
variance for grain yield in each stress environment did not differ from the
corresponding non-stress environment based on standard errors. Furthermore,
line x year interaction for grain yield in each stress environment was either
comparable to or significantly smaller than that in the corresponding non-stress
environment (Table 2).
TABLE 2. Components of variance and heritability estimates for grain yield, plant height, days to flowering of recombinant inbred lines for contrasting test environments
Environments |
Gen. var |
G x E var |
Heritability |
Grain yield |
|
|
|
Low fertility | 0.18±0.08** | 0.14±0.07** | 0.60±0.26 |
High fertility | 0.04±0.10 | 0.51±0.13** | 0.18±0.44 |
Rainfed | 0.16±0.08* | 0.21±0.08** | 0.54±0.28 |
Irrigated | 0.24±0.09** | 0.18±0.09** | 0.59±0.24 |
Plant height |
Low fertility | 0.83±0.19** | 0.09±0.02** | 0.96±0.22 |
High fertility | 0.86±0.19** | 0.05±0.02** | 0.97±0.21 |
Rainfed | 0.71±0.17** | 0.11±0.03** | 0.93±0.22 |
Irrigated | 0.89±0.19** | 0.05±0.02** | 0.97±0.21 |
Days to flowering |
Low fertility | 0.67±0.15** | 0.04±0.02* | 0.94±0.21 |
High fertility | 0.59±0.16** | 0.24±0.06** | 0.88±0.23 |
Rainfed | 0.49±0.13** | 0.13±0.05** | 0.86±0.23 |
Irrigated | 0.81±0.18** | 0.07±0.03** | 0.94±0.21 |
*, ** Significantly different from zero at P=0.05, and P=0.01 levels, respectively Although the genetic variances for plant height and days to flowering in
non-stress environments were slightly higher than those in the respective stress
environments, the differences were not significant statistically (P>0.05)
different. Heritability estimates for each trait in each contrasting
environment followed a similar trend as the genetic variance estimates (Table
2).
Blum et al. (1989) demonstrated that plant height and days to flowering
had marked effects on productivity when sorghum was grown under
drought stress. In our studies, however, the correlation of these
two traits with grain yields were very low (r<0.04) in both
stress and non-stress environments (Table 3). Genetic correlation
between low and high fertility environments for grain yield did not differ
significantly from zero (Table 4). Although the genetic correlation
between grain yields in rainfed and irrigated environments (rg=0.66) was more
than two times as large as its standard error, it was not very high either. Genetic
correlation between grain yields in rainfed and low fertility environments
was close to one. These results suggested that yield
responses of the RI lines in the two sets of contrasting environments, soil
fertility and moisture regime, were controlled by different sets of alleles. This
is in agreement with earlier studies (Atlin and Frey, 1990). By
contrast, the performances of inbred lines under rainfed and low fertility
environments were similar. Genetic correlations between contrasting
environments for plant height and days to flowering were significant and high
(Table 4).
TABLE 3. Correlation coefficients among grain yield, plant height and days to flowering inlow and high fertility as well as in rainfed and irrigated environments
Trait combinations |
Test environments |
| Low | High |
Rainfed |
Irrigated |
Yield with height | 0.35** | 0.27* | 0.02 | -0.02 |
Yield with flowering | -0.21* | 0.01 | -0.09 | 0.12 |
Height with flowering | -0.31** | -0.24* | -0.39** | -0.24* |
*, ** Significantly different from zero at P=0.05, and P=0.01 levels, respectively
TABLE 4. Genetic correlations between contrasting environments for grain yield, plant height, and days to flowering
Pairs of environments |
Genetic correlations |
|
Yield | Plant height | Days to floweing |
High and low fertility | 1.41±1.46 | 1.02±0.02 | 0.97±0.03 |
Irrigated and rainfed | 0.66±0.28 | 0.99±0.01 | 0.91±0.05 |
Rainfed and low fertility | 0.93±0.28 | 0.99±0.01 | 0.74±0.09 |
Predicted gain from indirect selection for grain yield in each non-stress
environment (high fertility and irrigated) was smaller than the predicted gain
from direct selection in the corresponding stress environment (low fertility
and rainfed), as indicated by the relative efficiency value of <1.0 (Table
5). Indirect selection for grain yields under rainfed condition
was found to be as efficient as direct selection under low soil fertility. Indirect
selection for plant height and days to flowering in each non-stress environment
produced as large a response in the respective stress environment as direct
selection in the stress environment.
TABLE 5. The ration of predicted response from indirect selection in non-stress environment to the response from direct selection in a stress environment (relative efficiency of indirect vs direct selection
Selection environment |
Response environmen |
Ratio of indirect to direct selection |
|
|
Grain yield |
Plant Height |
Days to flowering |
High fertility | Low fertility | 0.77 | 1.03 | 0.94 |
Irrigated | Rainfed | 0.69 | 1.01 | 0.95 |
Rainfed | Low fertility | -0.98 | 1.01 | 0.77 |
Indirect selection in low fertility environment was less effective
for improving grain yield under high fertility environment than direct selection
in high fertility environment (Table 6). In contrast, the
yield advances under low fertility environment resulting from indirect selection
in high fertility environment was nearly as high as that from direct selection
in low fertility environment. Selection based on the rank summation,
which included both low and high fertility environments, increased
yields significantly in these two contrasting environments. When
the index involved rainfed and low fertility environments, yield increases
of the selected lines was greater in low fertility environment than in high
fertility environment. Selection in the rainfed environment improved
yields significantly (P<0.05) in both rainfed and irrigated environments. On
the other hand, indirect selection in the irrigated environment failed to improve
yields under rainfed condition. Indirect selection for increased
yields based on the rank summation which involved both irrigated and rainfed
environments or rainfed and low fertility conditions were nearly as good as
the corresponding direct selections made in irrigated and rainfed environments
(Table 6).
TABLE 6. Mean grain yield of the best 10 lines selected in a single environment or a combination of environments by using the rank summation index which was expressed as a percentage of the overall mean of all the random lines tested in each response environment.
Selection environment |
Response environment |
|
Low fertility | High fertility |
Low fertility | 135** | 111 |
High fertility | 126** | 125** |
High + low fertility | 130** | 122** |
Rainfed + low fertility | 132** | 113** |
|
Rainfed | Irrigated |
Dryland | 131** | 124** |
Irrigated | 93 | 142** |
Irrigated + rainfed | 126** | 134** |
Rainfed + low fertility | 128** | 129** |
**Significantly higher than the overall mean of all the inbred lines (100%) at
P=0.01 level using LSD
To a breeder who is interested in developing inbred lines, the number of
superior lines which are common in the two contrasting environments is of great
importance. Of the best 10 lines selected under low fertility and
rainfed conditions using the rank summation, five lines were also present in
the top-ranking lines in high fertility and two lines were also among the top-ranking
lines under irrigated environments (Table 7). When the rank summation
involved two contrasting environments, between six and eight of the best 10
lines were common in both stressful and non-stressful conditions. It
is interesting to note that selection based on the rank summation
calculated from rainfed and low fertility environments identified at least
five of the top-ranking 10 lines in both stressful and non-stressful conditions.
TABLE 7. The number of top-ranking 10 recombinant inbred lines selected by the rank summation index from either a single environment or a combination of environments which were present in the top-ranking 10 lines of each contrasting response environment
Selection environment |
Response environment |
|
Low fertility | High fertility |
Low fertility | 10 | 5 |
High fertility | 5 | 10 |
High + low fertility | 7 | 8 |
Rainfed + low fertility | 7 | 5 |
|
Rainfed | Irrigated |
Rainfed | 10 | 2 |
Irrigated | 2 | 10 |
Irrigated + rainfed | 6 | 6 |
Rainfed + low fertility | 7 | 5 |
As sorghum is largely produced in nutrient and moisture deficit soils in
the semi-arid tropics, it is necessary to evaluate the genetic potential of
breeding materials to withstand these two stresses. Although, a
limited number of recombinant inbred lines were included in our studies, large
differences in grain yield among these lines were found in low fertility and
rainfed environments. Thus, the cross segregated for genes controlling
productivity in both nutrient deficient and moisture deficit soils. The
variation in grain yield was not correlated with the variation in plant height
and days to flowering, indicating that it should be possible to identify high
yielding lines specifically adapted to nutrient- and moisture- limited environments
in different maturity and height backgrounds.
One of the arguments for selecting in a favourable environment, even if improved
performance is sought for a stress environment, is that the former permits
greater genetic variance among lines with smaller year to year fluctuation
in genotypic performance than the latter (Blum, 1988; Frey, 1964; Srivastava et
al., 1983). The genetic and line x year interaction variance estimates
for grain yields in the high fertility and irrigated environments of our studies
did not indicate such trends. In fact, genetic variances for grain
yield in these two non-stressful conditions did not differ significantly (P>0.05)
from those in the corresponding stress conditions. The magnitude
of line x year interaction for grain yields in each non-stress environment
(high fertility or irrigated) was either comparable to or greater than that
in the respective stress environment (low fertility or rainfed). Even
if the genetic variance under non-stress condition was larger than the genetic
variance under stress condition, the differences for grain yields observed
in the absence of stress might be largely unrelated to the differences observed
in the presence of severe stress (Ceccarelli, 1987). Variation in productivity
of genotypes observed in the presence of drought stress may arise from differences
in anatomical, morphological, and physiological features (Blum et al.,
1989; Ludlow and Muchow, 1990). Furthermore, variation in the yielding
ability of genotypes in the presence of nutrient stress may be mediated by
differences in nutrient uptake, partioning of the nutrients into the grain,
and nutrient use efficiency (Muruli and Paulsen, 1981; Clark, 1982). Therefore,
detecting differences among genotypes for responses to stress by means of such
complex traits of adaptation will be difficult when stress is absent (Srivastava et
al., 1983).
Frey (1964) used the relative values of heritabilities in stress versus non-stress
conditions to predict progress from selection. Because the heritability
for each non-stress environment did not differ significantly from the heritability
for the corresponding stress environment in these studies, it was difficult
to use this criterion to predict the rate of progress from indirect selection
in non-stress environment that could be expressed in a stress environment. Others
also found no relationship between heritability estimates and the level of
productivity of test environments (Ceccarelli, 1989; Zavala-Garcia et al.,
1992). Ceccarelli (1989) pointed out that the difference in magnitude
of heritabilities between stress and non-stress conditions would be less important
than the extent to which differences expressed in one environment would be
maintained when the same set of genotypes were planted in another environment.
Indirect selection for increased yield under high fertility or irrigated
environment was found to be less efficient than direct selection in the corresponding
stress environment (low fertility or rainfed). However, selection
in each non-stress environment based on the rank summation index scores identified
lines which did well in the respective stress environment. Since
the genetic correlation was an overall inverse measure of the genotype x environment
interaction of the entire set of lines, some genotypes could interact very
little with contrasting environments notwithstanding the presence of a low
genetic correlation (Atlin and Frey, 1990).
Heterogeneity within lines derived from early segregating generations may
partly contribute to their broad adaptation to a range of contrasting environments
(Frey, 1964; Shabana et al., 1980). As the recombinant inbred
lines included in our studies were derived from the F6 generation, however,
heterogeneity within lines would be minimal at this level of inbreeding. Thus,
other mechanisms may confer broad adaptation of the recombinant inbred lines.
Since adaptation resulting from heterogeneity within lines cannot be fixed
(Frey, 1964; Shabana et al., 1980), the use of recombinant inbred lines
which are homogeneous may facilitate the transfer of genes for wide adaptation
intact from parents to their progenies.
Indirect selection for superior genotypes using a combination of low and
high fertility or rainfed and irrigated conditions identified at least six
of the top-ranking 10 lines in each contrasting environment. Even
when rainfed and low fertility environments were included in the
rank summation, at least half of the best 10 lines did very well in both low
and high fertility as well as in rainfed and irrigated environments. These
results indicated that greater gain in performance over contrasting environments
may be achieved by selecting for yield in more than one environment, rather
than by selecting in any single environment.
Our results suggested that selection for yield in moisture- and nutrient-
stress environments should be done directly in those environments. However,
the development of separate breeding programmes for moisture and nutrient stress
environments will be difficult and expensive. A more realistic approach
would be to screen breeding materials in plots which combine both drought and
nutrient stress. Srivastava et al. (1983) provided enough
evidence from selection experiments which were conducted in low fertility-dry farming
conditions that supported this approach. The strong genetic correlation
between rainfed and low fertility environments for yield and the common occurrence
of inbred lines which were top-ranking in both rainfed and low fertility
conditions in our studies provides additional support to this approach. To
achieve greater gain in performance in these two stress conditions, incorporating
parents with good performance under low input levels in crosses will be needed.
Because favourable growing seasons are occasionally encountered in sorghum
production environments, alternating selection in moisture and nutrient stress
with selection in favourable conditions may permit the identification of lines
which are adapted to both stressful and non-stressful conditions (Cercceralli,
1989).
REFERENCES
- Anderson, R.L. and Bancroft, T.A. 1952. Statistical Theory in
Research. McGraw-Hill Book Co., New York. 399pp.
- Atlin, G.N. and Frey, K.J. 1989. Predicting the relative effectiveness
of direct versus indirect selection for oat yield in three types of
stress environments. Euphytica 44:137-142.
- Atlin, G.N. and Frey, K.J. 1990. Selecting oat line for yield
in low productivity environments. Crop Science 30:556-561.
- Blum, A. 1988. Plant Breeding for Stress Environments. CRC
Press Inc., Boca Raton, FI. 223pp.
- Blum, A., Mayer, J. and Golan, G. 1989. Agronomic and physiological
assessments of genotypic variation for drought resistance in sorghum. Australian
Journal of Agricultural Research 40:49-61.
- Bramel-Cox, P.J., Baker, T., Zavala-Garcia, F. and Eastin, J.K. 1991. Selection
and testing environments for improved performance under reduced-input conditions. In:
Plant Breeding and Sustainable Agriculture: Considerations for Objectives
and Methods. Crop Science Society of America Special Publication
No. 18. pp. 29-56.
- Ceccarelli, S. 1987. Yield potential and drought tolerance
of segregating populations of barley in contrasting environments. Euphytica 36:265-273.
- Ceccarelli, S. 1989. Wide adaptation-How wide? Euphytica 40:197-205.
- Clark, R.B. 1982. Plant genotype differences to uptake, translocation,
accumulation, and use of mineral elements. In: Genetic Specificity
of Mineral Nutrition of Plants. Sariac, M.R. (Ed.), pp. 41-55. Serbian
Academy of Sciences and Arts, Beograd.
- Falconer, D.S. 1989. Introduction to Quantitative Genetics. 3rd Ed.
Longman Inc. New York. 438pp.
- Fox, P.N. and Rosielle, A.A. 1982. Reducing the influence of
environmental main-effects on patern analysis of plant breeding environments. Euphytica 31:452-645.
- Frey, K.J. 1964. Adaptation reaction of oat strains selected under stress
and non-stress environmental conditions. Crop Science 4:55-58.
- Hallauer, A.R. and Miranda, J.B, F.O. 1988. Quantitative Genetics
in Maize Breeding. 2nd Ed. Iowa State University Press, Ames. 468pp.
- Kawano, K. and Jennings, P.R. 1983. Tropical crop breeding-achievements
and challenges. In: Potential Productivity of Field Crops Under Different
Environments. International Rice Research Institute, Los Banos,
Laguna, Philippines. pp. 81-99.
- Lal, R. 1987. Managing the soils of sub-Saharan Africa. Science 236:1069-1076.
- Ludlow, M.M. and Muchow, R.C. 1990. A critical evaluation of
traits for improving crop yields in water-limited environments. Advances
in Agronomy 43:107-153.
- Mode, C.J. and Robinson, H.F. 1959. Pleiotropism and the genetic variance
and covariance. Biometrics 15:518-537.
- Muruli, B.I. and Paulsen, G.M. 1981. Improvement of nitrogen use efficiency
and its relationship to other traits in maize. Maydica 26:63-73.
- Rosenberg, N.J. 1984. The role of the metorologist and climatologist
in improving food production capabilities in the semi-arid regions. In: Agrometeorology
of Sorghum and Millet in the Semi-Arid Tropics. Proceedings
of the International Symposium, 15 - 20 November 1982, ICRISAT, India. 21pp.
- Seetharama, N., Nath, B. and Verma, P.K. 1984. Selection for
grain yield in low nitrogen fertility conditions. Cereal Res.
Com. 12:47-51.
- Shabana, R., Bailey, T. and Frey, K.J. 1980. Production traits
of oats selected under low, medium, and high productivity. Crop
Science 20:739-744.
- Srivastava, J.P., Jana, S., Gautam, P.L. and Niks, R.E. 1983. Parallel
selection: An approach to increase grain yield and stability. In: Proceedings
of the 6th International Wheat Genetics Symposium, Kyoto,
Japan. pp. 725-733.
- Zavala-Garcia, F., Bramel-Cox, P.J., Eastin, J.K., Witt, M.D. and Andrews,
D.J. 1992. Increasing the efficiency of crop selection for unpredictable
environments. Crop Science 32:51-57.
©2003, African Crop Science Society
|