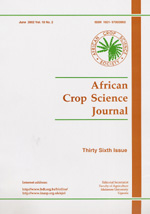
|
African Crop Science Journal
African Crop Science Society
ISSN: 1021-9730 EISSN: 2072-6589
Vol. 3, Num. 2, 1995, pp. 191-201
|
African Crop Science Journal, Vol. 3. No.2, pp. 191-201,
1995
Opportunities for biotechnology research on sorghum
Stalk rot and virus diseases
L.M. GIORDA and M.J. MARTINEZ ^1
EEA Manfredi-INTA, CP 5988, Cordoba, Argentina Laboratory of
Plant Pathology, Facultad de Ciencias, Agropecuarias, UNC, CC
508. Cordoba, Argentina
Code Number: CS95026
Size of Files:
Text: 45K
No associated graphics files
ABSTRACT
Although considerable progress has been made using conventional
technology in identification and characterization of viral and
stalk rot diseases of sorghum, there is still an incomplete
understanding of the genetics ofresistance, and many viral
diseases remain uncharacterized. Biotechnology IcDNA) will play
an increased role in virus detection and identification. and in
marker assisted selection in breeding for sorghum virus
resistance, and to discern the genetic basis of resistance to the
stalk rot complex quickly and more precisely. Biotechnology will
also aid in the identification of quantitative trait loci (QTL)
associated with specific Fusarium and Macrophomina
stalk rots responses and drought tolerance.
Key Words: cDNA. conventional breeding, drought tolerance,
marker assisted selection. virus resistance
RESUME
Bien que des progres considerables aient ete realises avec
la technique conventionelle d'identification et de
caracterization des maladies virales et de stalk rot de sorgho,
il reste a comprendre la resistance genetique eta caracteriser
beaucoup de maladies virales. La biotechnologie (DNA) jouera un
role prepoderant dans la detection et l'identification des
viroses, dans l'amelioration de la resistance aux virus a l'aide
de la selection, grace aux marqueurs et dans la determination
rapide et precise des bases genetiques de resistance au complexe
stalk rot. La biotechnologie aidera aussi a l'identification de
traits quantitatifs (QLT) au loci associes au fusarium et au
Macrophomia stalk rots eta la tolerance a la secheresse.
Mots Cles: DNA, amelioration conventionnelle, tolerance
a la secheresse, selection avec marqueurs, resistance a la
virose
VIRUS DISEASES
Sorghum viruses are distributed worldwide and cause economic
damage to the host. The first virus disease described in
sorghum was sugarcane mosaic, identified by Brandes and
Klaphaak (1923). Since then 23 viruses have been identified
within 12 groups; and in addition, other viruses or isolates
of potyvirus remain unclassified (Table 1). Seven viruses have
been experimentally transmitted to Sorghum spp. (Table
1 ). According to Peterschmitt etal. ( 1991 ), it
apl3ears that MStVsorg causes the most important virus disease
of sorghum in the Indian subcontinent, with an incidence of
10%. However, the potyvirus group are the most widespread and
prevalent viral path,ogens of sorghum (Giorda, 1993).
Although many viruses related to sorghum have been
isolated and characterized, several still have yet to be
identified.
TABLE 1. Viruses naturally and experimentally transmitted to
sorghum
Virus group Transmission
--------------------------------------------------------------
BROMOVlRUS*
Brome mosaic virus, BMV Nematode, beetle
CUCUMOVIRUS
Cucumber mosaic virus, CMV (Exp.) Aphid (NP)
FUROVIRUS*
Sorghum chlorotic spot virus, SChSV Fungus
Peanut clump virus, PCV Polymixa graminis
GEMINIVIRUS*
Maize streak virus, MSV (Exp.) (Ciccadulinaspp.)(LH)
LUTEOVIRUS
Barley yellow dwarf virus, BYDV MCDV Aphid (persistent)
Graminella nigrifrons,LH
Maize chlorotic dwarf virus, MCDV MCMV beetles
Maize chlorotic mottle virus, MCMV (Exp.) Aphid
(non-persistent)
POTYVIRUS
Johnsongrass mosaic virus, JGMV
Maize dwarf mosaic virus, MDMV
Sugarcane mosaic virus, SCMV
Sorghum mosaic virus, SrMV
Unclassified^1
MDMV-China
SOMV-India
MDMV-Venezuela
MDMV-D
MDMV-Honduras
SCMV-Honduras
MDMV-Africa
SCMV-Africa
Others
REOVlRUS*
Sugarcane Fiji Disease Perkinsiella
spp.(LH)
Maize rough dwarf virus, MRDV Dellacodes spp.(PH)
RHABDOVIRUS
Sorghum stunt mosaic virus, SSMV Graminella spp.(PH)
Maize mosaic virus, MMV Peregrinus spp.(PH)
SOBEMOVIRUS
Panicum mosaic virus, PMV (Exp.)
TENUIVIRUS*
Maize stripe virus, MStpV
Maize stripe virus, sorghum
-isolate, MStV-Song Peregrinus spp.
Sorghum red stripe virus, SRSV Peregrinus spp.
Rice stripe virus, RSV (Exp.) leafhopper
(?) Iranian wheat stripe virus, Unkanodes tanasijevici
IWhSV (Exp.)
Maize yellow stripe virus, MYSV (Exp.) (Delphasid)
leafhopper
(Cicadulina spp.)
Others^2
Sorghum yellow banding virus, SYBV
Sugarcane chlorotic mottle virus, SCMV
Johnsongrass chlorotic stripe mosaic virus, JCSMV
Sorghum mosaic disease, SMd
* Not mechanically transmitted
1^ Unclssified within the potyvirus class or subgroups.
^3 Unclassffied within the subgroups.
Antisera to some of them are not readily available and others
remain uncharacterized at the gene sequence level (Table 1).
Moreover, the genetic basis of resistance to virus diseases is
incompletely understood. Thus, several steps should be
considered sequentially or simultaneously, depending on the
host-pathogen system, the geographical area and the status of
the technology to assist in resolving these issues: (i)
biotechnological methods to assist virus detection and
identification. (ii) marker-assisted selection in breeding for
sorghum virus resistance. (iii) sorghum virus-engineered
resistance. and (iv) a cost/benefit analysis for the
application of biotechnological strategies to assist in
sorghum improvement.
Sorghum virus detection and identification. Serological
methods, using polyclonal antisera, have been widely applied
for many years for the detection and identification of plant
viruses. A limitation in the use of polyclonal antisera is the
inability to readily discriminate between strains of a virus.
One example is the potyvirus group.
Numerous strains which differ mainly in biological
properties, such as host range and/or pathogenicity, have been
reported on sorghum (Giorda, 1988). Attempts to identify and
classify distinct sorghum potyvirus and their strains have
frequently been hampered by the presence of variable
proportions of cross-reacting antibodies m the antisera. This
limitation has been overcome by the development of monoclonal
antibodies and other serological approaches such as
virus-specific antibodies using affinity chromatography
(Shukla et al., 1988; Ward and Shukla. 1991 ). A number
of other techniques can be used for sorghum virus detection
including ELISA and radioactive and nonradioactive nucleic
acid probes (Chu et al., 1989; Dietzgen et al.,
1994). However, some of these techniques are expensive and
labourintensive.
Other biotechnological approaches such as cDNA probes have
facilitated virus detection (Hamilton, 1992). Nucleic acid
hybridization is a powerful technique for detection of
complementary nuclei acid sequences and is being increaseingly
used for diagnosis of the potyvirus and other virus groups.
Henson and French (1993) reviewed the appplication of
polymerase chain reaction (PCR) to pathogen diagnosis.
PCR permits the amplification of specific DNA sequences
and offers several advantages compared to more
traditional methods of diagnosis (Bariana et al.,
1994). The reverse transcription polymerase chain reaction
(RT-PCR) assay simultaneously tests a sample in one tube for
the presence of different viruses (Bariana et al.,1994:
Smith and van de Velde, 1994). This new technique provides a
low cost tool for research into the incidence, ecology and
epidemiology of sorghum virus diseases.
RFLP-assisted breeding for sorghum virus resistance.
There are various ways in which damage from plant viruses
can be avoided. Each control method can be effective alone or
when combined with others. However, the most economical,
biologically safe and practical approach to control sorghum
virus diseases is the planting of resistant or tolerant
cultivars.
Genetics of resistance to sorghum virus diseases have been
described for the potyvirus group (Conde et al.. 1976;
Persley et al., 1977; Giorda, 1988). Considerable
variation among isolates of the subgroups MDMV, SCMV. JGMV and
SrMV have been demonstrated (Toler etaI., 1982; Tosic
et al., 1990; McKern et al., 1991).
In Australia, the inheritance of mosaic and necrotic
reaction was studied by Persley et al. (1977). Two
independent gene loci are involved in the control of the red
leaf (rlf and red stripe (N) reactions in sorghum.
Presence of the homozygous recessive allele rlf
conditions the expression of the necrotic red leaf reaction
following exposure of plants to low temperature.
Plants express only mosaic symptoms in the presence of the
dominant allele RLF and the absence of the N gene. The
presence of a single dominant host gene N results in the:
expression of necrotic red stripe symptoms. Conde et
al.(1976) described immunity conditioned by the Krish
gene. Krish resistance depends on a single gene K
with resistance (symptomless plants) dominant over
susceptibility. The relationship of the Krish
is monogenic, dominant and has been extensively used since
1971 in breeding programmes worldwide to develop cultivars
resistant to diflerent strains of JGMV, SCMV, SrMV and MDMV.
In 1985, red stripe symptoms occurred in a hybrid with Krish
resistance from one parent in Australia (Persley etal., 1987).
The appearance of isolates able to overcome Krish resistance
puts into questions the durability of their monogenic
resistance for potyvirus control.
Studies on the genetics of resistance to the sorghum
potyvirus group have attempted to fit the progeny of resistant
x susceptible crosses into symptom response classes. The
genetics of resistance to MDMV-A was based on disease severity
as measured by area under a disease progress curve (AUDPC) and
virus accumulation measured as ELISA values. The diallel
analysis for virus accumulation showed that the genetic
variation is mainly additive, and dominance for resistance to
virus accumulation accounted for the non-additive variation
(Giorda, 1988).
Field screening in areas of natural disease occurrence and
large-scale artificial inoculation procedures have contributed
to the improved MDMV/SCMV tolerance of commercial sorghum
cultivars.
A high density genetic linkage map is available for
sorghum (Pereira et al., 1994: Xu et al., 1994). Thus, RFLPs
could be used to assist in breeding and selection for MDMV-A
or SCMV-Jg (JGMV resistance even though it is monogenic
dominant, and thus selection is already relatively inexpensive
and straight forward. Should we use RFLPs to assist in the
selection for this type of trait with high heritability, that
is easy to evaluate and to improve through conventional means?
Although it is still far from "low tech", more effective
selection and fewer generations would be required in breeding
using RFLPs. It is valuable when a trait is recessive
(example, resistance to MDMVB or MDMV-V), difficult to assay
(viruses not mechanically transmitted, such as MStV-Sorg), and
in backcross breeding programmes to select simultaneously for
the desired chromosomal regions (for instance, genes
controlling disease resistance) and against unwanted
clucomosomal segments, such as those that might be introduced
while backcrossing from exotic germplasm or low yielding
parents with poor agronomic characteristics. This is very
important since it is possible to access genes from wild
relatives or photosensitive varieties where most sources of
resistance are found. Marker-assisted selection should be done
to pyramid genes into a single cultivar resistant to different
viruses or strains of the same pathogen.
The genetic basis of sorghum virus resistance as well as
screening for resistance is often confusing because natural
infection by different viruses or strains causes similar or
distinctive symptoms. Utilization of RFLPs would speed up
identification or detection of sources of resistance and it
could also be used to describe different types of gene
action.
Pratt et al. (1992) discussed the rationale involved in
deciding whether or not to use RFLPs to assist in the
improvement of yield and host plant resistance to maize virus
pathogens. They stated that the more difficult the traits are
to select by conventional methods, the stronger the argument
to use RFLP marker-assisted breeding. "Traits that can be
easily evaluated with conventional approaches are probably
more cost effective". Similar arguments might be applied to
sorghum improvement for virus resistance. Specific application
of RFLPs will depend on research priorities defined for each
region based on the constraints, economic development,
consumer and producer demand (food, industrial, or feed) and
defined market niches and policies.
Sorghum virus-engineered resistance. Coat
protein-mediated (CP) resistance has been successfully
demonstrated for viruses belonging to 10 groups including
potyvirus (Beachy et al., 1990; Beachy, 1993). This is the
most promising strategy being used in research on virus
resistance and is based on the phenomenon of crossprotection.
The protection actsonly againstrelated viruses showing a high
level of homology in the amino acid sequence of their coat
protein (Beachy et al., 1990).
CP-mediated resistance segregates as a dominant trait.
Genetic stability of this resistance is expected to be the same
as for any other gene from conventional breeding. For
instance, a single point mutation of a virus amino acid might
affect the level of resistance similarly to any other
monogenic resistance gene (Stiekema et al., 1993).
This argument disagrees with that of Zoeten (1991 ), who
indicated that the utilization of CP-mediated resistance to
control virus diseases could lead to the development of new
virus strains. The CP-mediated resistance is of a multiple
type: resistance to inoculation, to symptom expression, to
virus spread within the plant and to virus multiplication.
Stiekemaetal. (1993). speculating about durability of
CP-mediated resistance, indicated: "If the presence of viral
coat protein in each case blocks viral infection at different
levels e.g. the level of viral RNA dismantling, the level of
viral RNA replication and the level of systemic spread of the
virus in the plant, it is most likely that the resistance will
prove to be durable. In that case the virus has to mutate in
such a way that none of the three virus processes will be
inhibited by the presence of the coat protein. The durability
will be determined by the product of the probabilities of
circumvention by virus mutation of each of the individual
infection blocks".
Recently, Murry et al.(1993) developed transgenic maize
plants expressing MDMV-B CP. The more striking results are
that regenerated plants were resistant to MDMV-A, MDMV-B and
to mixed inoculations of MDMV and MCMV. This progress
encourages future research on sorghum virus diseases to
provide alternatives for strengthening or supplementing
existing genetic resistance. Since CP-mediated resistance
protects from related viruses, we can speculate on the
possibility of introducing MDMV-A CP into a high yielding
female sorghum line (A/B) without introducing undesirable
traits, and provide resistance to other virus or strains of
the potyvirus group infecting sorghum that share about 60%
homology in their amino acid sequence.
If the monogenic highly effective Krish gene lasted almost
15 years in Australia and remains effective elsewhere, the
question arises on how long will CP-mediated resistance will
last in transgenic sorghum plants?
Specific applications of the different biotechnologies for
control of sorghum virus diseases will depend on the local
problem, research facilities, and training capacities of
individual investigators. Forinstance, in Argentina, we have
characterized and identified MDMV-A. It is the most important
and prevalent virus in this sorghum growing region. Sources of
resistance have been detected and incorporated in the parental
lines. The use of RFLPs will enhance our breeding programme by
permitting the development of high yielding females with good
general combining ability and resistance to different diseases,
such as sorghum downy mildew, MDMV-A, and pests, such as
greenbug, by pyramiding the resistance genes from different B
or R line sources. However, sorghum is declining in importance
in Argentina as well as other Latin American countries.
Changes in production and consumption have led to pessimism
about the future impact of new technologies, and leading
to shift of potential investment in sorghum research to cash
crops such as soybean, maize, wheat, potato and cotton.
In other regions, such as different areas of Africa and
Central or South America. detection and identification of
sorghum viruses is a necessary step. Genetics of resistance to
several important virus diseases is lacking or incomplete
because they are difficult to work with under field
conditions. In these situations, biotechnology coupled with
traditional methods may greatly facilitate studies leading to
disease control.
In summary, molecularmarkers such as RFLPs are likely to
have a major impact on developing countries, providing
non-radioactive techniques and less expensive probing
techniques. "RFLP technology brings together molecular
genetics and classical plant breeding"(Young et al., 1992).
Its utilization for backcross breeding or for pyramiding
different resistance genes and defense genes, for instance,
would play an important role in sorghum crop improvement.
SORGHUM STALK ROT DISEASES
Stalk rots are widely distributed sorghum diseases and are
induced by several organisms. Macrophotnina phaseolina (Tass)
Goid and Fusarium moniliforme Sheld are the most prevalent
pathogens causing charcoal rot and Fusarium root and stalk
rot, respectively (Pande and Karunakar, 1992; Giorda et al.,
1995).
Yield losses from 15 to 60% may occur on susceptible
cultivars depending on the season and region (Mughogho and
Pande, 1984; Anahosur, 1992; Pande and Karunakar, 1992; Giorda
et at., 1995). Stalk rots account for an average loss of 4-8%
of the sorghum crop in USA (Claflin and Leslie, 1993).
However, good damage estimates from farmers' fields are
lacking and difficult to assess because of the association of
the disease with physiological stresses from water and heat or
from stress caused by greenbug teeding and different crop
management situations. Stalk rot is generally caused by more
than one pathogen under natural conditions (Pande and
Karunakar, 1992). The complex etiology of charcoal rot and
Fusarium stalk rot makes them challenging to work with.
Mihail et al. (1988) and Pande and Karunakar (1992) and
reviewed the present state of knowledge and progress on
different aspects of sorghum root and stalk rot diseases with
emphasis on charcoal rot. Fewer studies have been conducted on
the etiology, mode of action and host pathogen interactions of
Fusarium stalk rot.
Good control of these diseases can be obtained through
cultural practices of soil and water management techniques,
and by avoiding or decreasing greenbug damage by applying
insecticides or growing resistant cultivars (Doupnik. 1984;
Evangelista and Tangonan, 1991). However, this approach is
difficult to manage. considering that most of the area planted
with sorghum is rainfed and drought-prone.
Host resistance is the most practical long-term solution.
However, it has been difficult to identify stable sources of
resistance to charcoal rot or Fusarium root and stalk rot in
cultivars with good agronomic traits and resistance.
Both diseases need predisposing conditions (Pande and
Karunakar, 1992; Giorda etal., 1995) which affect the amount
of stalk rot and lodging, complicating the selection of
resistant genotypes as well as studies on host-pathogen
interactions. The source-sink relationship plays an important
role in the selection for resistance to lodging and to both
stalk rot diseases. Characters that aftect this relationship
such as grain yield and maturity must be considered when
selecting for those traits (Henzell et al., 1984;Rosenow,
1984). The size of the carbohydrate sink of the panicle is
important in determining the level of stress necessary to
induce stalk rot predisposition.
Different screening techniques, based mainly on senescence
or green leaf retention, lodging percentage, and extent of
pith degradation, have been used to select for stalk rot
resistance. However, the rating was not always done within
maturity and grain yield classes. Lack of consistent selection
pressure because of differences in the predisposing
physiological or biotic stresses, because of differences in
maturity or sink size, cause variations in the host response
and, consequently, influence the effectiveness of the
selection for disease reaction.
Several workers have studied the genetics of resistance to
F. moniliforme or M. phaseolina (Bramel-Cox et al., 1988;
Bramel-Cox and Claflin, 1989; Rosenow, 1992; Tenkouano et al.,
1993). Selection for resistance to M. phaseolina was done
indirectly through selection for drought tolerance. using
nonsenescence as the main criterion. Rosenow (1984) reported
significant correlations between nonsenescence, lodging
resistance and charcoal rot resistance in Texas, USA. Similar
results were obtained by Mughogho and Pande (1984) for
charcoal rot in India. Henzell et al. (1984) bred directly tot
lodging resistance and, thus. indirectly for stalk rot
resistance since lodging ("stem collapse") is the ultimate
effect of stalk rot in Australia. However, nonsenescence was
also identified by this author as the most important plant
character related to stalk rot (M. phaseolina and F.
moniliforme), lodging, and postfloral drought resistance.
Maunder (1984) also mentioned: "selection for stiff stalk and
drought tolerance, especially of the nonsenescent type,
combined with high yield will be more productive than breeding
for charcoal rot resistance alone".
Fusarium stalk rot resistance has also been indirectly
screened for by selecting individual plants or populations
with lodging resistance, greenbug resistance or
nonsenescence characteristics. Henzell et al. (1984) suggested
that the reaction of genotypes to M. phaseolina and F.
,tonil~fonne may be inherited independently and that there may
be a correlation between lodging resistance and resistance to
M. phaseolina but not to F. moniliforme.
Bramel-Cox et al. (1988) reported that the inheritance of
resistance to F. moniliforme and M. phaseolina based on
artificial inoculation techniques is controlled by a
multiple-locus complex with distinct heterotic patterns. The
expression of resistance appeared to be dependent on the
environment (both organisms) or maturity (M. phaseolina).
Duncan and de Millano (1995) and Rosenow (1992) indicated
recessive/intermediate inheritance patterns for both charcoal
rot and Fusarium stalk rot. Recent studies (Tenkouano etal.,
1992; 1993) on the genetics of nonsenescence and charcoal rot
resistance in sorghum determined that nonsenescence was
regulated by dominant and recessive epistatic interactions
between two nonsenescence-inducing loci and a third locus with
modifying effects. This was also true for charcoal rot
resistance. The authors concluded that "nonsenescence alone
can not account for, and should not be used as the sole
breeding criterion for, resistance to charcoal rot in
sorghum".
MIGHT BIOTECHNOLOGY SPEED UP SORGHUM IMPROVEMENT FOR STALK
ROTS RESISTANCE?
Fusarium root and stalk rot. Little is known about the
host-pathogen interactions, however, progress was made by
developing Fusarium stalk rot-tolerant cultivars screened for
characters other than resistance to the pathogen perse. Three
main characters have been mentioned in the literature as
associated with tolerance to Fusarium stalk rot: lodging
resistance, nonsenescence and greenbug resistance (Henzell et
al., 1984; Mughogho and Pande, 1984; Rosenow, 1984; Giorda
et al. 1994). The first two might be considered phenotypic
expressions of postflowering drought tolerance. Drought
tolerance is a complex trait influenced by the interactive
effects of many genes. Ejeta (1993) has identified several
"quantitative trait loci" (QTL) associated with pre- and post-
flowering stress tolerance.
Since complex traits are involved, it might be possible to
discern the genetic basis of the resistance quickly and more
precisely with RFLPs. Tolerance to moisture stress under post-
flowering drought conditions is manifested by a stay-green
(nonsenescence) phenotype. Most of the genotypes with enhanced
drought tolerance often show limited yield potential.
Several reports have summarized the application of
different molecular markers to tag useful genes and to
determine the gene action (Paterson et al., 1988; Tanksley et
al., 1989; Keim et al., 1990; Ueng et al., 1992; Hulbert,
1995; Jones and Canada, 1994). Based on these observations,
efforts should concentrate on identifing QTL loci associated
with specific Fusarium stalk rot responses under uniform
conditions for disease development. In this way, once traits
(postflowering drought tolerance and Fusarium root and stalk
rot resistance genes) are tagged, it might be possible to use
RFLPs to improve high yielding susceptible lines by assembling
combinations of these desirable genes.
Studies on Fusarium spp. taxonomy and:strain
differentiation are in progress using biotechnological
methods (Leslie, 1991, 1993; Mansuetus, 1993; Zhang et al.,
1994). Similarly, molecular markers are being used to tag
drought tolerance traits (Tunistra et al., 1993; Rosenow,
1994). The application of molecular genetics with classical
plant pathology and plant breeding should work together to
develop new strategical approaches to solve these problems.
Charcoal rot. Although much progress has been made in
breeding for charcoal rot resistance by using the
nonsenescence trait ( Rosenow, 1984; Pande and Karunakar,
1992), new information indicates that additional criteria for
resistance to charcoal rot should be used (Tenkouano et al.,
1993 ). Mechanisms of resistance to M. phaseolina are still
unknown, although research on those traits is being conducted
(Frederiksen, 1993). The relationship of nonstructural
carbohydrate (NSC) partitioning and charcoal rot resistance in
sorghum was investigated by Tenkouano et al. (1992). These
authors suggested that high yielding cultivars resistant to M.
phaseolina could be developed since the developing grain could
not be identified as the cause or the beneficiary of stem NSC
exhaustion. So far, most of the unaffected hybrids tolerant to
charcoal rot yield nearly as well as the unaffected
susceptible ones. These observations are encouraging for
improving sorghum for this trait.
Different biotechnological approaches are currently being
used to detect variability among M. phaseolina isolates (Chase
et al., 1994). Knowledge ofpathogen variability would be
useful in breeding programmes. In general, similar approaches
to Fusarium root and stalk rot resistance might be used in
studies on control of charcoal rot. Plant response to disease
by induction of a large array of host response genes and
modification of the expression of these genes could lead to
enhanced disease resistance. This approach has recently been
investigated and might also constitute a strategy to improve
resistance to charcoal rot (Cui etal., 1994; Nelson and
Bushnell, 1994).
CONCLUSIONS
In summary, F. moniliforme and M. phaseolina have different
requirements of temperature and soil moisture conditions
fordisease establishment and development. However, diseases
caused by both pathogens are induced if stress conditions
occur at anthesis or post-flowering. Complex traits are
involved in the genetics of resistance to both pathogens,
while selection procedures are troublesome and the results
sometimes inconsistent. Effective and faster improvements of
these traits may be done by applying molecular technologies.
Attempts should be made to tag the different genes associated
with the resistance to sorghum stalk rot diseases and to use
linked RFLPs, for instance, to pyramid these genes into a
single line. Resistant gene combinations may contribute to the
durability of resistance ( Kolmer et al., 1991; Mundt, 1991;
de Wit and van Kan, 1993).
REFERENCES
Anahosur. K.H. 1992. Sorghum diseases in india: knowledge
and research needs. In: Sorghum and Millets Diseases:A Second
World Review. de Milliano, W.A.J., Frederiksen. R.A. and
Bengston, G.D. (Eds.), pp. 45-46. ICRISAT, Patancheru 502324,
A.P., India.
Bariana, H.S., Shannon, A.L., Chu, P.W.G. and Waterhouse, P.M.
1994. Detection of five seedborne legume viruses in one
sensitive multiplex poIymerase chain reaction test.
Phytopathology 84:1201 -1205.
Beachy, R.N. 1993. Virus resistance through expression of coat
protein genes. In: Biotechnology in Plant Disease Control.
Chet, I. (Ed.), pp. 89-104. Wiley-Liss, Inc., New York, NY,
USA.
Beachy, R.N., Loesch-Fries, S. and Tunmer, N.E. 1990. Coat
protein mediated resistance against virus infection. Annual
Review of Phytopathology 28:451-474.
Bramel-Cox, P.J. and Claflin, L.E. 1989. Selection for
resistance to Macrophomina phaseolina and Fusarium moniliforme
in sorghum. Crop Science 29:1468-1472.
Bramel-Cox, P.J., Stein, I.S., Rodgers, D.M. and Claflin, L.E.
1988. Inheritance of resistance to Macrophomina phaseolina
(Tassi) Goid. and Fusarium moniliforme Sheldon in sorghum.
Crop Science 28:37-40.
Brandes. E.W. and Klaphaak, P.J. 1923. Cultivated and wild
hosts of sugarcane or grass mosaic. Journal of Agricultural
Research
24:247-262.
Chase, T.E., Yiang, Y. and Mihail, J. 1994. Molecular
variability in Macrophomina phaseolina. Phytopathology
84:1149 (Abstract).
Chu, P.W.G., Waterhouse. P.M., Martin, R.R. and Gerlach, W.G.
1989. New approaches to the detection of microbial plant
pathogens. Biotechnology and Genetic Engineering
Review 7:45-111.
Claflin, L.E. and Leslie, J.F. 1993. Agroecology and
biotechnology of stalk rot pathogens of sorghum and millet.
Pages 2-9. In: INTSORMIL Annual Report, USA. 260 pp.
Conde, B.D., Moore, R.F., Fletcher, D.S. and Teakle, D.S. 1976.
Inheritance of the resistance of Krish sorghum to sugarcane
mosaic virus. Australian Journal of Agricultural Research
27:45-52.
Cui, Y., MagiIl, C., MagiIi, J. and Frederiksen, R.A. 1994.
Molecular markers for defense response genes in sorghum.
Phytopathology 84:1112 (Abstract).
de Wit, J.M.J. and van Kan, J.A.L. 1993. Is durable resistance
against fungi attainable through biotechnological procedures?
In: Durability of Disease Resistance. Jacobs, T. and Parlcvliet,
J.E. (Eds.), pp. 57-70. Kluwer Academic Publishers, London, U.K.
Dietzgen, R.G., Wu, Z. and Teycheney, P.Y. 1994.
Dioxigenin-labeled cDNA probes for the detection of two potyvirus
infecting peanut (Arachis hypogea). Plant Disease 78:708711.
Doupnik, B., Jr. 1984. Cultural and biological control of root
and stalk rot diseases of sorghum. In: Sorghum Root and Stalk
Rots, A Critical Review. Mughogho, L.K. and Rosenberg, G. (Eds.),
pp. 201-208. ICRISAT, Patancheru 502324, A.P., India. 267 pp.
Duncan, R.R. and de MiIliano, W.A.J. 1995. Plant disease control
in sorghum and pearl millet. In: Disease Analysis Through
Genetics and Molecular Biology: Interdisciplinary Bridges to
Improved Sorghum and Millet Crops. Leslie, J.F. and Frederiksen.
R.A. (Eds.), pp. 23-53. Iowa State University Press, Ames, IA,
USA.
Ejeta, G. 1993. Development and enhancement of sorghum germplasm
with sustained tolerance to drought, striga, and grain mold.
Pages 122128. In: INTSORMIL Annual Report. USA. 260 pp.
EvangeIista, C.C. and Tangonan, N.G. 1991. Root and stalk rot
incidence in non-senescent sorghum genotypes and theirchemical
control. Research and Development Philippines 67:27-28.
Frederiksen, R.A. 1993. Disease control strategies for
sustainable agricultural systems. Pages 23-30. In:
INTSORMILAnnuaIReport. USA. 260 pp.
Giorda, L. 1988. Interactions of Virus Accumulation, Disease
Severity, and Disease Incidence as Heritable Indicators of Host
Resistance 10 MDMV-A and Their Influence on Yield. Ph.D.
Dissertation, Texas A&M University, College Station, TX, USA. 219
PP.
Giorda, L. 1993. Situacion actual de la investigacion de las
enfermedades virosicas del sorgo. Aspectos epidemiologicos y
manejo. Actas del seminario Internacional sobre Enfermedades de
Mafz y Sorgo. C .I. Tibai tara. Santa Fe de Bogota, Colombia
27-29 Enero.
Giorda, L., Martinez, M.J. and Chulze, S. 1995. Fusarium root and
stalk rot in Argentina. In: Disease Analysis Through Genetics and
Molecular Biology: Interdisciplinary Bridges to Improved Sorghum
and Millet Crops. Leslie, J.F. and Frederiksen, R.A. (Eds.), pp.
185-193. Iowa State University Press, Ames, IA, USA.
Hamilton, R.I. 1992. The use of monoclonal antibodies and cDNA
for detection of plant viruses. In: Biotechnology: Enhancing
Research on Tropical Crops in Africa. Thottappilly. G., Monti,
L.M., Mohan Raj, D.R. and Moore, A.W. (Eds.), pp. 297-303.
IITA/CTA, Ibadan, Nigeria. 376 pp.
Henson, JM and French, R. 1993. The polymerase chain reaction and
plant disease diagnosis. Annual Review Phytopathology 31:81 -
109.
Hertzell, R.G., Dodman. R.L., Done, A.A., Brengman, K.L. and
Mayers, P.E. 1984. Lodging stalk rot, and root rot in sorghum in
Australia. In: Sorghum Root and Stalk Rots: A Critical Review.
Mughogho, L.K. and Rosenberg, G. (Eds.), pp. 225-236. ICRISAT,
Patancheru 502324, A.P., India.
Hulbert, S.H. 1995. Molecular markers and the construction of
genetic maps. In: Disease Analysis Through Genetics and
Molecular Biology: Interdisciplinary, Bridges to Improved Sorghum
and Millet Crops. Leslie, J.F. and Frederiksen, R.A. (Eds.), pp.
95-113. Iowa State University Press, Ames, IA, USA.
Jardine, D.J. 1986. Stalk Rots of Corn and Sorghum. KSU
Cooperative Extension Service Bulletin L-741, 4pp.
Jones, R.W. and Canada, S. 1994. Electrophoretic karyotype
analysis and mapping of an endoglucanase gene from Macrophomina
phaseolina. Phytopathology 84:/146. (Abstract).
Keim, P.B., Diers, W. and Shoemaker, R.C. 1990. Genetic analysis
of soybean hard seededness with molecular markers. Theoretical
Applied Genetics 79:465-469.
Kolmer, J.A., Dych, P.L. and RoeIfs A.P. 1991 An appraisal of
stem and leaf rust resistance in North American hard red spring
wheats and the probability of multiple mutations to virulence in
populations of cereal rust fungi. Phytopathology 81:237-239.
LesLie, J.F. 1991. Mating populations in Gibberella fujikuroi:
(Fusarium section Liseola). Phytopathology 81:1058-1060.
Leslie, J.F. 1993. Fungal vegetative compatibility. Annual Review
Phytopathology 31:127-150.
Mansuetus, S .B .A. 1993. Mating Populations and Vegetative
Compatibility Groups Within Gibberella fujikuroi (Fusarium
Section Liseola) on Sorghum in Tanzania. Ph.D Dissertation,
Texas A&M University, College Station, TX, USA. 96 pp.
Maunder, A.B. 1984. Breeding for stalk rot resistance as a
component of acceptable agronomic performance. In: Sorghum Root
and Stalk Rots:A Critical Review. Mughogho, L.K. and Rosenberg,
G. (Eds.), pp. 219-224. ICRISAT, Patancheru 502324, A.P.,
India.
McKern, N.M., Shukla. D.D., Toler, R.W., Jensen, S.G., Tosic, M.,
Ford, R.E., Leon, O. and Ward, C.W. 1991. Confirmation that the
sugarcane mosaic virus subgroup consists of fourdistinct
potyvirus by using peptide profiles of coat protein.
Phytopathology 81:1025-1029.
Mihail, J.D., Young, D. J. and Alcorn, S .M. 1988. Macrophomina
phaseolina: A plant pathogen of concern in arid lands. In: Arid
Land Today and Tomorrow. Whitehead, E.E., Hutchinson. C.F.,
Timmerman, B.W. and Varody, R.G. (Eds.), pp. 1305-1310. Tucson,
AZ, USA.
Mughogho, L.K. and Pande. S. 1984. Charcoal rot of sorghum. In:
Sorghum Root and Stalk Rots: A Critical Review. Mughogho. L.K.
and Rosenberg, G. (Eds.), pp. 11-24. ICRISAT, Patancheru 502324.
A.P., India.
Mundt, C.C. 1991. Probability of mutation to multiple virulence
and durability of resistance gene pyramids: further comments.
Phytopathology 81:240-242.
Murry, L.E. Elliott, L.G., Capitant, S.A., West, J.A., Hanson,
K.K., Scararia, L., Jolmston. S., DeLuca-Flaherty, C., Nichols,
S., Cunanan, D., Dietrich, ES., Mettler, I.J., Deward, S.,
Warnick, D.A., Rhodes, C., Sinibaldi, RM. and Brunke, K.J. 1993.
Transgenic corn plants expressing MDMV strain B coat protein are
resistant to mixed infections of maize dwarf mosaic virus and
maize chlorotic mottle virus. Bio/Technology I 1:1559-1564.
Nelson, A.J. and Bushnell, R. 1994. A rapid method for evaluation
of defense gene constructs for their utility in enhancing disease
resistance. Phytopathology 84:1147 (Abstract).
Pande. S. and Karunakar, R.I. 1992. Stalk rots. In: Sorghum and
Millets Diseases:A Second World Review. de Milllano, W.A.J.,
Frederiksen, R.A. and Bengston, G.D. (Eds.), pp. 219-234.
ICRISAT. Patancheru 502324, A.P., India. 370 pp.
Paterson. A.H., Lauder, E.S.. Hewitt, J.D., Peterson, S.,
Lincoln, S.E. andTanksley, S.D. 1988. Resolution of quantitative
traits into Mendelian factors by using acomplete linkage map of
restriction fragment length pol ymorphisms. Nature
335:721-726.
Peteira, M.G., Lee, M., Bramel-Cox, P.J. and Woodman, W.L. 1994.
Construction of a complete genetic linkage map in sorghum based
on RFLPs. In: Use of MolecularMarkers in Sorghum and Pearl Millet
Breeding for Developing Countries. Witcombe, J.R. and Duncan,
R.R. (Eds.), pp. 6-8. Proceedings of an ODA Plant Science
Research Programme Conference, Norwich, U,K. 126 pp.
Persley, D.M., Greber, R.S. and Henzell, R.G. 1987. Isolates of
sugarcane mosaic virusjohnson grass strain infecting Krish
resistant grain sorghum genotypes in Australia. Sorghum
Newsletter 30:72-73.
Persley, D.M., Moore, R.F. and Fletcher D.S. 1977. The
inheritance of the red leaf reaction of grain sorghum to
sugarcane mosaic virus infection. Australian Journal of
Agricultural Research 28:853-858.
Peterschmitt. M., Ratna, A.S., Sacks, W.R., Reddy, D.U.R. and
Mughogho, L.K. 1991. Ocurrence of an isolate of maize stripe
virus on sorghum in India. Annals of Applied Biology
118:57-70.
Pratt, R.C., McMullen. M.D. and Louie, R. 1992. RFLP
marker-assisted breeding for maize virus resistance. In:
Biotechnology: Enhancing Research on Tropical Crops in Africa.
Thottappilly, G., Monti, LM, Mohan. D.R., and Moore, A.W. (Eds.),
pp. 247-254. IITA/ CTA, Ibadan, Nigeria. 376 pp.
Rosenow, D.T. 1984. Breeding for resistance to root and stalk
rots in Texas. In: Sorghum Root and Stalk Rots: A Critical
Review. Mughogho, L.K. and Rosenberg, G. (Eds.), pp. 209-218.
ICRISAT, Patancheru 502324, A.P., India. 267 pp.
Rosenow, D.T. 1992. Using germplasm from the world collection in
breeding for disease resistance. In: SorghumandMilletsDiseases:
A SecondWorldReview. de Milliano, W.A.J., Frederiksen, A. and
Bengston, G.D. (Eds), pp. 319-324. ICRISAT, Patancheru 502324,
A.P. India. 370 pp.
Rosenow, D.T. 1994. Evaluation for drought and disease resistance
in sorghum for use in molecular marker-assisted selection. In:
Use of Molecular Markers in Sorghum and Pearl Millet Breeding for
Developing Countries. Witcombe, J.R. and Duncan, R.R. (Eds.), pp.
27-31. Proceedings of an ODA Plant Science Research Programme
Conference, Norwich. U.K 126 pp.
Shukla, D.D., Strike, P.M., Tracy, S.L., Gough. K.H. and Ward,
C.W. 1988. The N and C termini of the coat protein of potyvirus
are surface located and the N terminus the major virus-specific
epitopes. Journal of General Virolagy 69: 1497-1508.
Smith, G.R. and Van de VeIde. R. 1994. Detection of.sugarcane
mosaic virus and Fiji disease virus in diseased sugarcane using
the polymerase chain reaction. Plant Disease 78:557-561.
Stiekema, W.J., Visser, B7 and Florack. D.E.A. 1993. Is durable
resistance against viruses and bacteria attainable via
biotechnology? In: Durability of Disease Resistance. Jacobs, T.
and Parlevliet, J.E. (Eds.), pp. 71-81. Kluwer Academic
publishers. London, U.K.
Tanksley, S.D., Young, N.D.. Paterson, A.H. and Bonierbab, MW.
1989. RFLP mapping in plant breeding: New tools for an old
science. Bio/Technology 7:257-264
Tenkouano, A., Miller, F.R., Frederiksen. R.A. and Rosenow, D.T.
1993. Genetics of nonsenescence and charcoal rot resistance in
sorghum. Theoretical Applied Genetics 85:644-648.
Tenkouano, A., Miller, F.R. and Frederiksen, R.A. 1992.
Nonsenescence and charcoal rot resistance in sorghum: linkage but
not pleiotropy. Sorghum Newsletter 33:33.
Toler, R.W., Rosenow, D.T., Riccelli. M. and Mena, H.A. 1982.
Variability of Venezuelan isolate of maize dwarf mosaic virus in
sorghum. Plant Disease 66:849-850.
Tosic, M., Ford, R.E., Shukla, D.D. and Jilka, J. 1990.
Differentiation of sugarcane, maize dwarf, johnsongrass. and
sorghum mosaic viruses based on reactions of oat and some sorghum
cultivars. Plant Disease 74:40-552.
Tunistra, M., Goldsbrough, P., Grote, E. and Ejeta, G. 1993.
Identification and RAPD mapping of quantitative trait loci
associated with drought tolerance in sorghum. Agranomy Abstract
p. 184.
Ueng, P.P., Bergstrom, G.C., Slay, R.M., Geiger, E.A., Shaner,
G. and Scharen, A.L. 1992. Restriction fragment lengh
polymorphism in the wheat gl ume blotch fungus, Phaeosphaeria
nodorum. Phytopathology 82:1302-1305.
Ward, C.W. and Shukla, D.D. 1991. Taxonomy of potyviruses:
Current problems and some solutions. Intervirology 32:269-296.
Xu, G.W., MagiIi, C.W. and Frederiksen, R.A. 1994. Construction
of an RFLP linkage map of Sorghum bicolor (L) Moench. In: Use of
Molecular Markers in Sorghum and Pearl Millet Breeding for
Developing Countries. Witcombe. J.R. and Duncan, R.R. (Eds.), pp.
9. Proceedings of an ODA Plant Science Research Program
Conference. Norwich, U.K. 126 pp.
Young, N.D., Menancio-Hautea, D., Fatokun, C.A. and Danesh, D.
1992. RFLP technology, crop improvement. and international
agriculture. In: Biotechnology: Enhancing Research on
Tropical Crops in Africa. Thottappilly. G., Monti, L.M., Mohan
Raj, DR. and Moore, A.W. (Eds.) pp. 221-230. 1ITA/CTA, Ibadan,
Nigeria.
Zhang, D., Qiu, J., Shelby, R.A. and Tuzun, S. 1994. DNA
amplification fingerprinting of isolates from different
vegetative compatibility groups of Fusarium moniliforme.
Phytopathology 84:1146. (Abstract).
Zoeten. G.A. 1991. Risk assessment: Do we let history repeat
itself? Phytopathology 81:585586.
Copyright 1995 African Crop Science Society
|