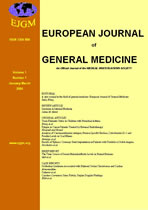
|
European Journal of General Medicine
Medical Investigations Society
ISSN: 1304-3897
Vol. 5, Num. 2, 2008, pp. 57-63
|
European Journal of General Medicine, Vol. 5, No. 2, 2008, pp. 57-63
Plasma And Cerebrospinal Fluid Homocysteine, Nitric Oxide And Malondialdehyde Levels In Acute Ischemic Stroke: Possible Role Of Free Radicals In The Development Of Brain Injury
Recep Aygül1, Dilcan Kotan1,, Abdulkadir Yıldırım2, Hızır Ulvi1, Fatih Akçay2
Atatürk University, Medical Faculty, Departments of Neurology1 and Biochemistry2, Erzurum, Turkey
Correspondence: Dr Dilcan Kotan, MD, Ataturk Universitesi, Tip Fakültesi,
Noroloji Anabilim Dali, 25240 Erzurum, Turkey
Phone: 904422361212/1122, Fax: 904422361301
E-mail:dilcan@atauni.edu.tr
Code Number: gm08012
Aim: Free radical mechanisms may play an important role in brain ischemia / reperfusion injury. The generation of reactive oxygen species by homocysteine (Hcy) or nitric oxide (NO) metabolism might be involved in the induction of lipid peroxidation, as indicated by malondialdehyde (MDA) formation. The purpose of this study was to investigate the behavior of Hcy, NO and MDA in plasma and cerebrospinal fluid (CSF) within 4 days after ischemic stroke onset.
Methods: The levels of NO, Hcy and MDA were determined in plasma and CSF on the 3rd or 4th days after the onset of ischemic stroke in 29 patients (12 men and 17 women) and in 13 healthy controls (6 men and 7 women) of comparable age and gender.
Results: The plasma MDA, NO and Hcy levels were significantly higher in the stroke patients, while mean MDA, NO and Hcy levels in CSF showed a significant increase in the cases as compared to controls (p<0.01). There were no gender-specific differences in the plasma or CSF MDA, NO and Hcy concentrations. A significant negative correlation was found between duration of diabetes with CSF NO (r=-0.63, p<0.001). The low Glasgow Coma Scale (GKS) was negatively correlated with lethal outcome, (r=-0.76, p<0.001), but it did not correlate significantly with any of the measured parameters.
Conclusion: These data support that free radical mechanisms may play a role in the development of brain injury following ischemic stroke. It was suggested that the elevated Hcy may be an important risk factor for acute ischemic cerebral injury.
Key words: Ischemic stroke, oxidative brain injury, nitric oxide, malondialdehyde, homocysteine
INTRODUCTION
Stroke is the third most common cause of death in industrialized countries, and is a major cause of severe physical disability (1, 2). Ischemic stroke accounts for 70 to 80% of all strokes. Cerebral infarction may be due to primary thrombosis in an artery or to occlusion of a vessel by an embolus (3). Independently from the mechanism responsible for the vessel occlusion, ischemia causes a cascade of events that eventually lead to neuronal damage and death (4, 6).
It is now generally accepted that oxidative stress means over-generation of reactive oxygen species (ROS) and lipid peroxidation plays an important role in the pathogenesis of neuronal damage induced by ischemia-reperfusion (5). The presence of high levels of polyunsaturated fatty acids in the membrane lipids of the brain is a source for lipid peroxidation reactions (5, 6). Products of lipid peroxidation, such as malondialdehyde (MDA) and 4-hydroxynonenal, were found to be increased in subjects with thrombotic or cardioembolic ischemic stroke than in controls (6-9).
ROS production was increased in the brain during ischemia and this process was detectable even in plasma (6). Excitotoxic stimulation of superoxide and nitric oxide (NO) production in ischemia-reperfusion leads to formation of highly reactive products, including peroxynitrite and hydroxyl radical, which are capable of damaging lipids, proteins and DNA (9). In this way, NO and ROS act independently as well as cooperatively to induce neuronal death in acute ischemic stroke (10).
In recent years, numerous studies have identified a strong, independent and dose-related association between elevated Hcy levels and vascular diseases, including stroke (11- 18). The exact mechanisms responsible for this association is still under investigation. High Hcy levels produce complex changes within the blood vessel wall. These changes include oxidative stress, proinflammatory effects such as expression of inducible NO synthase, platelet activation, and endothelial injury (15, 17, 19). Endothelial dysfunction is hypothesized to be caused by the potency of Hcy to interfere with the action of endothelial vasodilators such as NO (14). It is postulated that NO is scavenged by superoxide anion generated in the metabolic pathway of Hcy (16). When NO formation is reduced, excessive Hcy may further damage the endothelium by generation of ROS (15, 17), thereby increasing lipid peroxidation (18). Furthermore, high Hcy-induced oxidative stress may occur as a result of decreased expression and/or activity of key antioxidant enzymes as well as increased enzymatic generation of superoxide anion (19). Superoxide anion and hydroxyl radical generated during oxidation of Hcy initiate lipid peroxidation, an effect that occurs both at the endothelial cell surface as well as within lipoprotein particles in plasma (20).
The objective of the present investigation was to obtain evidence for the involvement of free radical-induced oxidative damage by measuring the plasma and cerebrospinal fluid (CSF) levels of a variety of oxidants in patients with ischemic stroke on the 3rd or 4th days after the accident: Hcy, MDA and NO.
MATERIALS AND METHODS
The oxidants’ activities in plasma and CSF were measured sequentially in 29 patients with acute ischemic stroke who were admitted to the Department of Neurology, Ataturk University, within 3 days after onset of symptoms. Informed consent was obtained from all participants or their relatives before lumbar puncture. The results were compared with those from 13 healthy subjects of comparable age and gender. All patients had demographic and medical data collected including history of hypertension, diabetes mellitus, smoking, alcohol and drug intake, and cardiovascular diseases.
The stroke was initially diagnosed on the basis of neurological examination and brain CT scan or MRI. Patients were clinically evaluated on hospital day one and at discharge using the Glasgow Coma Scale (GCS) (21). The diagnosis was also confirmed by brain CT or MRI at the third day after stroke onset. Functional outcome at discharge was assessed using the Glasgow Outcome Scale (GOS) (22). The GOS evaluates clinical outcome with a functional status scale comprising of 5 items. One point represents the best score (recovery) and five points the worst result (death). On the basis of clinical and neuroradiological criteria, it was possible to distinguish patients as having lacunar or nonlacunar syndromes, including total anterior, partial anterior and posterior syndromes. Patients with subarachnoid hemorrhage, cerebral hemorrhage, renal failure, hepatic disease and severe medical illness were not included in the study. All patients were treated similarly during hospitalization. Therapy was based on the preservation of essential life functions and the prevention of secondary medical complications.
Heparinized fasting venous blood samples were taken on the 3rd or 4th days of the attack for determination of plasma Hcy, MDA and NO levels. The blood samples were centrifuged and the plasma samples obtained were stored at –80°C until analysis. Patients underwent lumbar puncture for diagnostic purpose, for instance to exclude subarachnoid or cerebral hemorrhage. Control CSF samples were obtained from 13 age-matched controls who underwent lumbar puncture to give intrathecal anesthetic drugs during orthopedic surgery. None of these subjects had evidence of systemic or central nervous system diseases, such as ischemic or hemorrhagic stroke. Also, CSF samples were immediately stored at -80°C until the time of analysis. Hcy, NO and MDA determinations in plasma and in CSF were performed with blinding to clinical findings and to stroke outcome.
The levels of plasma and CSF Hcy were determined by using high-performance liquid chromatographic method (23). MDA levels were determined spectrophotometrically by a method similar to that described in the literature (24). NO levels were measured using the Griess reagent by method Moshage et al (25).
Statistical Analysis
The statistical analysis was performed with Statistical Package for the Social Sciences software for Windows (SPSS, version 10.0, Chicago, IL). The results are reported as the mean (95% CI) and median (range). Due to the small number of cases, the statistical and correlation analyses were carried out with the Mann–Whitney U-test and Spearman’s rank correlation test coefficient, respectively. Statistical significance was set at p<0.05.
RESULTS
The mean volume of the cerebral infarction was 32.5±10.7 cm3 (range 12.6-57.8). The baseline characteristics of ischemic stroke patients and controls are shown in Table 1. Twenty-six patients had nonlacunar syndromes and 3 had lacunar infract. Fifteen patients (51.7%) were hypertensive, 4 (13.8%) had diabetes mellitus and 7 (24%) had hypercholesterolemia. None of the subjects studied had a history of alcohol abuse. Indices of renal and hepatic function were within the normal ranges in all subjects. According to the evaluation of stroke outcome at discharge, 6 patients died. The causes of mortality were a direct result of infarction (2 cases), cardiac arrest (2 cases), pulmonary embolism (1 case) and unknown (1 case).
Table 1. Baseline characteristics of stroke cases and controls
Parameter |
Controls (n:13) |
Cases (n:29) |
p |
Age (mean±SD,y) |
58.1±6.8 |
61.9±10.4 |
ns |
Sex (male/female) |
6/7 |
12/17 |
ns |
Cigarette Smoking |
4 (30.8%) |
9 (31%) |
ns |
Hypertension |
- |
15 (51.7%) |
- |
Hypercholesterolemia |
- |
7 (24%) |
- |
Diabetes Mellitus |
- |
4 (13.8%) |
- |
ECG evidence of atrial fibrillation |
- |
5 (17%) |
- |
Coronary heart disease |
- |
7 (24%) |
- |
ns: Non-significant
As shown in Table 2, the plasma MDA, NO and Hcy levels were significantly increased in stroke patients, as compared to controls (p<0.01, for all). Mean MDA, NO and Hcy levels in CSF were higher in cases compared with controls (p<0.01) in Table 3. There were no gender-specific differences in the plasma or CSF MDA, NO and Hcy concentrations.
Table 2. Fasting plasma homocysteine, nitric oxide and malondialdehyde concentrations in cases and controls
|
Cases (n:29) |
Control (n:13) |
p |
Homocysteine, µmol/L |
|
|
|
Median (range) |
16.2 (8.2-42.4) |
8.3 (5.9-16.4) |
0.000 |
Mean (95% CI) |
19.1 (15.5-22.6) |
9.3 (7.3-11.3) |
Nitric Oxide, µmol/L |
|
|
|
Median (range) |
24.9 (15.0-35.2) |
22.1 (10.6-27.3) |
0.013 |
Mean (95% CI) |
25.6 (23.9-27.2) |
21.5 (18.8-24.3) |
Malondialdehyde, µmol/L |
|
|
|
Median (range) |
14.5 (5.7-29.1) |
11.6 (4.1-15.5) |
0.004 |
Mean (95% CI) |
14.3 (12.8-15.9) |
10.6 (8.2-12.9) |
Table 3. Cerebrospinal fluid homocysteine, nitric oxide and malondialdehyde levels in cases and controls
|
Cases (n:29) |
Control (n:13) |
p |
Homocysteine, µmol/L |
|
|
|
Median (range) |
0.45 (0.22-1.0) |
0.28 (0.10-0.56) |
0.006 |
Mean (95% CI) |
0.53 (0.44-0.63) |
0.29 (0.20-0.39) |
Nitric Oxide, µmol/L |
|
|
|
Median (range) |
9.2 (7.4.0-13.5) |
8.5 (5.4-9.9) |
0.015 |
Mean (95% CI) |
9.2 (8.8-9.7) |
8.1 (7.4-8.9) |
Malondialdehyde, µmol/L |
|
|
|
Median (range) |
11.8 (7.1-18.0) |
8.0 (4.3-12.7) |
0.001 |
Mean (95% CI) |
11.8 (10.6-13.2) |
8.3 (7.0-9.6) |
The infarction volume, GOS and GCS did not correlate significantly with any of the measured individual plasma or CSF oxidant concentrations. None of these parameters were associated to the age of the study subjects or with smoking status (duration of smoking x number of cigarettes/d). A significant negative correlation was found between low GCS scores and lethal outcomes (r=-0.76, p<0.001) and between duration of diabetes with CSF NO (r=-0.63, p<0.001). Also, there were a positive correlation between the CSF MDA with plasma NO levels (r=0.40, p<0.05).
DISCUSSION
We measured plasma and CSF lipid peroxidation to investigate oxidative stress. Lipid peroxidation is the most studied biologically relevant, free radical reaction. Our findings of raised MDA in plasma and CSF of ischemic stroke patients are in good accordance with other studies (6-9, 26-28). Since MDA is a specific marker of lipid peroxidation, these data suggested that free radical production was increased in the brain during ischemia and that this process was detectable even in plasma (6) and CSF. Once generated, free radicals can react with all the cellular macromolecules leading to lipid peroxidation, DNA and protein oxidation (30). Our data support the concept of oxidative stress as a factor in the pathogenesis of ischemic stroke. There is strong evidence for involvement of free radicals and lipid peroxidation in the pathophysiology of acute ischemic stroke (6-9, 26-28). There are several possible reasons for increased lipid peroxidation in ischemic stroke. First the brain cellular membrane is very rich in polyunsaturated fatty acid side chains, which are especially sensitive to free radical attack (29). Then it has a low content of antioxidant enzymes, such as catalase and glutathione peroxidase, while it contains a significant amount of iron despite its iron binding capacity is not very high. Iron ions are known to stimulate free radical generation (30). Meanwhile, it has been claimed that lipid peroxidation products are a key mediator of neuronal apoptosis induced by oxidative stress (32). Antioxidants that suppress lipid peroxidation protected against apoptosis induced by oxidative insults (10, 31). Several drugs with antioxidant activity such as tirilazad mesylate, a lipid peroxidation inhibitor (31) and BN 80933, a dual inhibitor of neuronal NO synthase and lipid peroxidation (10) have been shown to be able to reduce infarct volume and neurological impairment after brain ischemia.
This study demonstrates that NO levels in plasma and CSF are higher in patients with ischemic stroke than in healthy controls. The significant elevation of NO is most probably due to the induction of NO synthase by the effect of ischemic condition. Such an observed increase in CSF NO levels in our patients matches the observation of Castillo et al (33) and raise NO levels in plasma seems to be consistent with El-Kossi et al study (8). Castillo et al (33) have previously shown that significantly increased NO in CSF of ischemic stroke patients. Those studies indicate that in patients who suffer from ischemic stroke there is a strong induction of NO production within the CNS which is hypothesized to contribute to oxidative stress and subsequent neuronal damage. In Castillo et al study, it was also shown that increased NO in CSF of stroke patients is associated with a greater brain injury and early neurological deterioration (33). A significant negative correlation was found between duration of diabetes with CSF NO. The data support previously published data suggesting that central nitrergic neuronal pathways could be involved in regulating and maintaining glucose homeostasis (34). However, we found no correlation between infarction volume or neurological deterioration with NO levels. The lack of correlation between NO and infarction volume or early neurological deterioration may be due to the small sample size. The role of NO in ischemic brain injury is protective or destructive depending on the stage of evolution of the ischemic process and on the cellular compartment producing NO (35). Reaction of NO with superoxide causes formation of peroxynitrite that initiates lipid peroxidation via reaction of lipids with its decomposition products (hydroxyl radical and nitrogen dioxide) (9).
To our knowledge, this is the first study supporting at the clinical setting an important role of both plasma and CSF Hcy elevation in acute ischemic stroke. We have found a significant increase of Hcy in CSF and in plasma of patients studied on the 3rd or 4th days from stroke onset in comparison to controls. Our findings corroborate those of other investigators (11-13) indicating high homocysteine levels as a risk factor for ischemic stroke. CSF amino acid levels are only 40% of plasma levels, being actively transported to CSF through an active mechanism at choroid plexuses level. Because amino acids do not diffuse freely across the blood–brain barrier, it can be hypothesized that increased CSF Hcy concentrations indicate a decreased catabolism (36) or an impairment of blood-brain barrier in CNS in acute stroke. There was no correlation between Hcy levels and patient age, blood pressure, glucose values, smoking status, duration of diabetes mellitus or hypertension. It was suggested that high homocysteine levels may be an independent risk factor for acute cerebral vascular disease.
There is growing evidence that high Hcy levels contribute to the pathogenesis of ischemic stroke. Numerous studies and reviews have identified a strong and independent association between elevated Hcy and vascular disease, including stroke (11, 19). Hcy is postulated to cause ischemic stroke via various mechanisms. However its action mechanism and its role in the acute phase of stroke have not been enlightened, yet. High homocysteine levels are believed to cause atherogenesis and thrombogenesis via endothelial damage, vascular smooth muscle proliferation, and coagulation abnormalities (15, 19). It produces changes in structure and function of cerebral blood vessels. Oxidative stress appears to play an important role in mediating such changes (19). High homocysteine levels induced by acute methionine loading, produces impaired autoregulatory responses in older humans (14).
High homocysteine levels induced oxidative stress may occur as a result of decreased expression and/or activities of key antioxidant enzymes as well as increased enzymatic generation of superoxide anion (19). Superoxide anion and hydroxyl radical generated during oxidation of Hcy initiate lipid peroxidation, an effect that occurs both at the endothelial cell surface as well as within lipoprotein particles in plasma (20). On the other hand, sulfhydryl compounds, such as Hcy, are believed to attenuate endothelial production of bioactive NO through the reaction of superoxide anion generated during their auto-oxidation with NO, resulting in the formation of the adduct peroxynitrite (37). Thus, despite the increased production of NO after Hcy exposure, less bioactive NO is available owing to its inactivation by superoxide anion produced during Hcy’s oxidation (19). These mechanisms likely account for the results of Lentz and colleagues in which endothelium-dependent vasodilator responses were attenuated following Hcy exposure in a primate model (38). Zhang et al (39) determined that the infusion of Hcy with copper inhibits NO-related vasodilator responses by scavenging of NO in a rat model. This may be one of the mechanisms by which Hcy predisposes to ischemic stroke (39).
Several potential limitations of our report are those present in like previous reports. Even though patients were selected consecutively, confounding can never be fully eliminated. Numbers of stroke cases and controls were also small. Although etiologic subtypes of ischemic stroke have been shown to affect Hcy levels (11), differences among stroke subtypes were not assessed in our study due to the small sample size. The initial aim of our study was to compare control subjects with acute stroke patients without to be taken into consideration of etiologic subtypes. When the results were analyzed and statistically significant differences between patients and control subjects were found, we examined the hypothesis that plasma and CSF Hcy levels may increase in the acute phase in stroke cases. It is now claimed that Hcy level would be increased gradually after stroke about 10% in the first week, and the elevated Hcy might be a consequence, rather than a cause, of the stroke (40). These results await confirmation from other studies.
In conclusion, we found that increased NO, MDA and Hcy levels in plasma and CSF of patients with acute ischemic stroke. All the studies mentioned above support the hypothesis that Hcy plays a role in the pathogenesis of ischemic stroke. It is possible that a higher oxidative damage is a consequence of the higher oxidant levels in the acute phase of stroke.
REFERENCES
- De Freitas GR, Bogousslavsky J. Primary stroke prevention. Eur J Neurol 2001;8:1-15
- Broderick J, Brott T, Kothari R, et al. The Greater Cincinnati/Northern Kentucky Stroke Study: preliminary first-ever and total incidence rates of stroke among blacks. Stroke 1998;29:415–21
- Sudlow CL and Warlow CP. Comparable studies of the incidence of stroke and its pathological types: results from an international collaboration. International Stroke Incidence Collaboration. Stroke 1997;28:491–99
- Fisher M and Schaebitz W. An overview of acute stroke therapy. Past, present, and future. Arch Intern Med 2000;160:3196-206
- Love S. Oxidative stress in brain ischaemia. Brain Pathol 1999;9:119–31
- Cherubini A, Polidori C, Benedetti C, Ercolani S, Senin U, Mecocci P. Association between ischemic stroke and increased oxidative stres. 2nd Virtual Congress of Cardiology - FAC, Argentina, Sep 1-Nov 30,2001
- Re G, Azzimondi G, Lanzarini C, Bassein L, Vaona I, Guarnieri C. Plasma lipoperoxidative markers in ischemic stroke suggest brain embolism. Eur J Emerg Med 1997;4:5–9
- El-Kossi MMH, Zakhary MM. Oxidative Stress in the context of acute cerebrovascular stroke. Stroke 2000;31:1889-97
- Warner DS, Sheng H, Haberle IB. Oxidants, antioxidants and the ischemic brain. J Exp Biol 2004;207:3221-31
- Chabrier PE, Auguet M, Spinnewyn B, et al.BN 80933, a dual inhibitor of neuronal nitric oxide synthase and lipid peroxidation: a promising neuroprotective strategy. Proc Natl Acad Sci USA 1999;96:10824-29
- Eikelboom JW, Hankey GJ, Anand SS, Lofthouse E, Staples N, Baker RI. Association between high homocysteine and ischemic stroke due to large- and small-artery disease but not other etiologic subtypes of ischemic stroke. Stroke 2000;31:1069-75
- Giles WH, Croft JB, Greenlund KJ, et al. Total homocysteine concentration and the likelihood of nonfatal stroke: results from the Third National Health and Nutrition Examination Survey, 1988–1994. Stroke 1998;29:2473–77
- Bostom AG, Rosenberg IH, Silbershatz H, et al. Nonfasting plasma total homocysteine levels and stroke incidence in elderly persons: the Framingham Study. Ann Intern Med 1999;131:352–55
- Chao CL, Lee YT. Impairment of cerebrovascular reactivity by methione-induced hyperhomocysteinemia and amelioration by quinapril treatment. Stroke 2000;31:2907–11
- Thambyrajah J, Townend JN. Homocysteine and atherothrombosis — mechanisms for injury. Eur Heart J 2000;21:967–74
- Rosengarten B, Osthaus S, Auch D, Kaps M. Effects of acute hyperhomocysteinemia on the neurovascular coupling mechanism in healthy young adults. Stroke 2003;34:446-51
- Misra HP. Generation of superoxide free radical during the autooxidation of thiols. J Biol Chem 1974;249:2151–55
- Jones BG, Rose FA, Tudball N. Lipid peroxidation and homocysteine induced toxicity. Atherosclerosis 1994;105:165–70
- Faraci FM, Lentz SR. Hyperhomocysteinemia, oxidative stress, and cerebral vascular dysfunction. Stroke 2004;35:345-47
- Heinecke JW, Rosen H, Suzuki LA, Chait A. The role of sulfur-containing amino acids in superoxide production and modification of low-density lipoprotein by arterial smooth muscle cells. J Biol Chem 1987;262:10098-103
- Teasdale G, Jenett B. Assessment of coma and impaired consciousness: a practical scale. Lancet 1974;2:81–3
- Jenett B, Bond M. Assessment of outcome after severe brain damage: a practical scale. Lancet 1975;1:480–4
- Fiskerstrand T, Refsum H, Kvalheim G, Ueland PM. Homocysteine and other thiols in plasma and urine: automated determination and sample stability. Clin Chem 1993;39: 263–71
- Slater TF: Overview of methods used for detecting lipid peroxidation. Methods Enzimol 1984;105:283-93
- Moshage H, Kok B, Huizenga JR, Jansen PL. Nitrite and nitrate determinations in plasma: A critical evaluation. Clin Chem 1995;41: 892-96
- Demirkaya S, Topcuoglu MA, Aydin A, Ulas UH, Isimer AI, Vural O. Malondialdehyde, glutathione peroxidase and superoxide dismutase in peripheral blood erythrocytes of patients with acute cerebral ischemia. Eur J Neurol 2001;8:43-8
- Sharp PC, Mulholland C, Trinic KT. Ascorbate and malondialdehyde in stroke patients. Ir J Med Sci 1994;163:488–91
- Selakovic VM, Jovanovic MD, Jovicic A. Changes of cortisol levels and index of lipid peroxidation in cerebrospinal fluid of patients in the acute phase of completed stroke. Vojno Pregl 2002;59:485-91
- Watson BD. Evaluation of concomitance of lipid peroxidation in experimental models of cerebral ischemia and stroke. Prog Brain Res 1993;96:69–95
- Halliwell B. Reactive oxygen species and the central nervous system. J Neurochem 1992; 59:1609-23
- Hall ED, McCall JM, Means ED. Therapeutic potential of the lazaroids (21-aminosteroids) in acute central nervous system trauma, ischemia and subarachnoid hemorrhage. Adv Pharmacol 1994;28:221-67
- Mattson MP, Culmsee C, Yu ZF. Apoptotic and antiapoptotic mechanisms in stroke. Cell Tissue Res 2000;301:173-87
- Castillo J, Rama R, Dávalos A. Nitric Oxide–Related brain damage in acute ischemic stroke. Stroke 2000;31:852-58
- Shankar R, Zhu J-S, Ladd B, Henry D, Shen H-Q, Baron AD. Central Nervous System Nitric Oxide Synthase Activity Regulates Insulin Secretion and Insulin Action. J Clin Invest 1998;102:1403–12
- Iadecola C. Bright and dark sides of nitric oxide in ischemic brain injury. Trends Neurosci 1997;20:132-9
- Serot JM, Béné MC, Faure GC. CSF homocysteine, CSF folates and choroid plexus. Neurobiol Aging 2003;24:627-8
- Jia L, Furchgott RF. Inhibition by sulphydryl compounds of vascular relaxation induced by nitric oxide and endothelium-derived relaxing factor. J Pharmacol Exp Ther 1993;267:371-8
- Lentz SR, Sobey CG, Piegors DJ, Bhopatkar MY, Faraci FM, Malinow MR, Heistad DD. Vascular dysfunction in monkeys with diet-induced hyperhomocysteinemia. J Clin Invest 1996;98:24-9
- Zhang F, Slungaarg A, Vercellotti GM, Iadecola C. Superoxide-dependent cerebrovascular effects of homocysteine. Am J Physiol 1998;274:1704–11
- Howard VJ, Sides EG, Newman GC, Cohen SN, Howard G, Malinow MR, Toole JF. Changes in plasma homocysteine in the acute phase after stroke. Stroke 2002;33: 473–78
Copyright 2008 - Medical Investigations Society
|