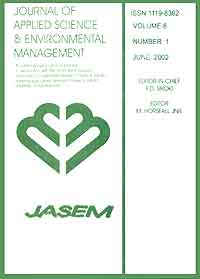
|
Journal of Applied Sciences and Environmental Management
World Bank assisted National Agricultural Research Project (NARP) - University of Port Harcourt
ISSN: 1119-8362
Vol. 15, Num. 1, 2011, pp. 155-160
|
Journal of Applied Science & Environmental Management Vol. 15 No.1, March
2011, pp. 127-133
Tanzanian Botanical Derivatives in the Control of Malaria
Vectors: Opportunities
and Challenges
Charles Kihampa
Department of Environmental Science and Management, Ardhi University, P. O. Box 35176, Dar es Salaam, Tanzania Email: kihampa@yahoo.com
Code Number: ja11026
ABSTRACT
This paper report on assessment of the chemicals derived from Tanzanian botanical
resource as a viable source of safe, environmentally friendly and low cost
mosquitocidal agents, but has yet to be developed into simple blends and formulations
to be used in malaria control campaigns. Selection of bioactive plant species
were based on random screening, phytochemical targeting, ethnobotanical survey,
chemotaxonomic approach and targeted screening approach. The crude extracts
from leaves, stem and root barks, and pure isolated compounds from over 20
different Tanzania plant species have been established to possess antimosquito
activities. The bioassay experiments were based on cytotoxicity screening in
BST followed by mosquitocidal assays of larvicidal, tarsal contact and repellency
bioassays. The activities were characterized to be larvicidal, growth disruptors,
insecticidal and repellents. Seasonal variations, geographical locations, isolation
of small amounts of pure compounds, limits in the availability of essential
facilities such as NMR spectroscopy, MS and X-ray diffraction analysis for
structural elucidation, stereochemical and conformational assignments, have
been realised to be among the impediments in the search and establishment of
new and biodegradable botanical natural products. The
results suggest that the investigated plant species are promising as mosquitocidal
agents and could be useful leads for the development of new insecticide products.
@JASEM
Malaria is the most prevalent and dangerous of the
tropical and subtropical diseases, being transmitted
from person to person by the bite of an infected
female Anopheles mosquito. The disease ranks high
among the major killer diseases which are endemic to
the tropics. As high as 90 million clinical cases of
malaria have been reported annually in Africa and
children are mostly affected (Sachs, 2002). Tanzania
is probably one of the worst hit malaria endemic
countries in Africa, having areas with highest
transmission. The disease is a leading health problem
in the country and a threat to every one of the
estimated 32 million people (94 % of the population)
living in areas where transmission is possible (MOH,
2006). For several years now, the world community
has been investing a lot of effort to eradicate malaria
globally but these attempts have so far been
unsuccessful particularly in most developing
countries. Insecticides, chemotherapy and campaigns
to keep the environment clear of mosquito breeding
sites are so far the only means of combating malaria
(The Wellcome Trust, 1999).
Over the past 20 years or so several researchers have
embarked on the search for insecticides that would be
useful in the control of malaria transmitting
mosquitoes and other insect pests. Campaigns aimed
at eradicating malaria transmitting mosquitoes were
initially intensified after World War II, immediately
after the discovery of the insecticide chemical DDT
(Nkunya, 2004). The initial campaigns were very
successful in eradicating malaria-transmitting
mosquitoes in many parts of the world, particularly
when these programmes were coupled with the
application of pyrethroid insecticides (Kakkilaya,
2007). However, due to vastness and poor
communication infrastructure these campaigns could
not yield the expected results in most countries in
Africa, Asia and Latin America (Yekutiel, 1980).
Furthermore, poor economies, environmental
concerns and development of physiological resistance
by many insects including mosquitoes against DDT
and its analogues led to the termination of the
mosquito eradication programmes. Nonetheless,
naturally occurring pyrethrins and their synthetic
analogues continue to be applied in mosquito control
programs at household scales. So far these
compounds have not been shown to have adverse
effects to humans since they are readily
biodegradable. Likewise insects have not developed
resistance against these insecticides (Yamaguchi,
1990).
Plants are highly vulnerable to predation by
herbivorous animals, insects, fungi and other
microorganisms within the ecosystem. Therefore,
plants synthesize a variety of chemically diverse
secondary metabolites, at least in part in response to
selection pressures against predation and/or attack by
herbivores and other microorganisms. In this regard
plants found in the tropical climates have been
considered to be viable sources of chemical agents
with effective properties and hence would be used to
protect the plants against predation. This is believed
to be the case since tropical conditions are quite
challenging to the plants’ survival, as the plants are
threatened by a myriad of organisms that thrive under
tropical climatic conditions. Therefore, through
evolutionary transformations, tropical plants must
have evolved the ability to metabolise toxic compounds that can interfere with
the basic
biochemistry of the predatory or harmful organisms,
such compounds are therefore suitable candidates for
the development of medicinal and/or pest control
agents (Nkunya, 2004; Sukumar et al 1991).
The application of herbal remedies in insect control either by repelling, killing and/or attracting insects, ticks and mites have been known since ancient times. The insecticidal plants have been used in various forms to control mosquitoes, for example Ancient peoples used smoke from burning cattle or goat dung to drive out mosquitoes from their caves or huts before sleeping. Later on, certain herbs and barks of some trees were added to the smoldering fire to enhance the repellent action of smoke. The Ainu people of Hokkaido, Japan and Micmac Indians of Newfoundland wore legginings of sedge, bark or cloth to reduce insect biting nuisance, which is concentrated around the lower legs. The Chinese had many prescriptions of repellents against mosquitoes, among other blood-sucking flies (Curtis, 1991). During World War II, the military used aromatic oils like citronella, bergamot, eucalyptus, peppermint, turpentine and spirit of camphor in various formulations to repel biting insects. Many plants used in traditional medicines have in fact been found to be sources of safe insecticides, from which several present day insecticides have been developed. The isolation of a highly potent and safe insecticide, pyrethrins, from the flower bud of pyrethrum (Chrysanthemum cinererifolium), rekindled efforts to search for insecticides from bioactive plants, although such efforts had remained dormant after DDT and synthetic insecticides was discovered in 1970 (Yamaguchi, 1990).
There has been a growing interest in recent years to develop antimosquitocidal and other insecticides from bioactive plant species. In the most extensive programme to screen plant extracts for insecticidal activity reported in 1997, over 2,000 plant species from 60 families were discovered to possess insecticidal properties, from which several compounds that are effective against insects have been isolated. Indeed extracts or even pure compounds from plant families such as Annonaceae, Asteraceae, Cyperaceae, Ebeneceae, Euphorbiaceae, Lamiaceae, Lauraceae, Leguminosae and Meliaceae have been established to be promising in the control of mosquitoes and other insect pests, being effective at various stages of the insect developmental growth (Dev and Koul, 1997).
METHODOLOGY
Selection and collection of the plant materials: In the selection of
plant species for pharmacological and insecticidal screening, five approaches
have been considered to be effective. These include random screening which
involves collection of all plant species from a particular study area, phytochemical
targeting that aimed at the collection of all members of the plant´s
family known to be rich in bioactive compounds, ethnobotanical survey that
is based on traditional uses of the plant(s), chemotaxonomic approach that
is based on plants having similar constituents which may be in different families,
and targeted screening of specific parts of a plant such as the seeds, barks,
roots, leaves and other parts. Among these approaches, it is generally acknowledged
that sampling based on ethnobotanical approach gives a greater percentage yield
of bioactive compounds over the other methods, although targeted and random
screening of plants and their extracts for activity have also yielded excellent
results (Farnsworth, 1966; Khafagi and Dewedar, 2000).
Different plant parts such as roots, stems, leaves and fruits were collected with the help of botanists from different areas in Tanzania. Information on the antimosquito potency of targeted plants was based on ethnobotanical information, existing literature and interview to local community members during study visits. Plants collected were authenticated at the Herbarium of the Department of Botany, University of Dar es Salaam where voucher specimens are preserved.
Bioassays: In most cases before embarking into mosquitocidal bioassays, samples were first screened for possible bioactivity in the cytotoxicity test on brine shrimp test (BST) which is quick and cheap. The materials which were active in the BST assays were found to be active in mosquitocidal activity as it is described by Joseph et al 2004. The mosquito bioassays at different stages followed the protocol described by WHO (WHO, 1996).
Brine shrimp lethality test: The brine shrimp lethality test (BST) was used to predict the presence, in the extracts and isolated pure compound, for cytotoxicity activity, which in this respect is correlated to the antimosquito potency (Meyer et al., 1982). Solutions of the extracts and pure compounds were made in DMSO or distilled water at varying concentration and were incubated in triplicate vials with the brine shrimp larvae. Ten shrimp larvae were placed in each of the duplicate vials. Control brine shrimp larvae were placed in a mixture of seawater and DMSO only. After 24 h, nauplii were examined against a lighted background, and the average number of survived larvae in each triplicate was determined. The mean percentage mortality was plotted against the logarithm of concentrations, and the concentrations killing 50 % of the larvae (LC50) was determined graphically (Meyer et al., 1982).
Bioassays against mosquito larvae: Screening of the candidate samples
was performed by first exposing the larvae in distilled water treated with
a series of four dilutions, at 1.0, 0.5, 0.25 and 0.125 mg/L. Then 20 late
3rd or early 4th stage larvae were
used per cup with each concentration tested in triplicate. Mortality was observed
after 24 h
and the graph of percentage mortality rates against the logarithms of concentrations
was plotted for LC50 determinations (WHO, 1996). Control experiments were performed
by exposing the larvae in a mixture of 1 mL of alcohol diluted to 1 L with
distilled water.
Mosquitocidal assays by tarsal contact methods on mosquito bed nets: Solutions
of the test sample at different dosages were impregnated onto three mosquito
nets (1 m2) that were placed in three cones (“treated sets”).
Three other mosquito nets to which only solvent had been impregnated were used
as controls. After the solvent had dried from the mosquito nets, 10 non-bloods
fed female mosquitoes were placed into each of the cones through a hole. The
mosquitoes were allowed to be in contact with the nets for 3 min, then transferred
into paper cups and observed for 1 h after which a glucose solution was applied
into each paper cup to serve as food and the mosquitoes were observed after
24 h. The knockdown effect and mortality of the mosquitoes was recorded and
the results analysed graphically.
Mosquito bioassay by tarsal contact with deposits on filter paper: The tests conducted by applying a series of dosages by dipping the filter paper (Whatman No. 1) in 50 ml solutions of the sample dissolved in alcohol. The papers were air dried for 24 h and then inserted into WHO tubes for testing. A group of 10 blood-fed female mosquitoes were placed in a tube containing treated filter paper for 3 min. The mosquitoes were then returned to holding tubes for determination of average mortality relationship after 24 h. LC50 values were determined from the graph of mortality against logarithms of concentration.
Mosquito repellency bioassays: The bioassays were carried out in a dark room with red light as the only source of illumination. The room temperature and humidity were artificially set using a heater and humidifier to mimic the host feeding conditions for the female An. gambiae mosquitoes (Temp. 27-35 °C and relative humidity > 65%).
All the repellency tests were carried out on 5-7 days old mosquitoes that had
been starved overnight, but previously fed on 6 % glucose solution. Six human
volunteers were used in the repellency bioassays. They were not allowed to
use lotions, perfumes, oils or perfumed soaps on the day of the bioassay. Various
test solutions of the compounds were prepared in acetone. The highest concentration
was 1% (0.01 g/ml). Therefore, the bioassayed doses were of 0.000015, 0.00015,
0.0015, 0.015 and 0.15 mg/cm2. A test solution (0.5 ml) was dispensed on the right forearm
of a volunteer from the wrist to the elbow. The rest of the hand was covered
with glove to make it unattractive to the mosquitoes. Acetone (0.5 ml) was
dispensed on the left forearm, to act as control. The arms were swapped regularly
to eliminate any bias. The control arm was introduced into the cage immediately
after releasing the 25 insects and kept there for 3 min. The mosquitoes that
had landed on that arm during the test duration were recorded. The treated
arm was then introduced into the cage and kept there for 3 min. The number
of mosquitoes that landed on the treated arm was also recorded. The screening
was done sequentially starting with the lowest dose (0.001%) and ending with
the highest one (1%). Each concentration was screened using a fresh batch of
mosquitoes. After the bioassay of each concentration, the arms were washed
with bar soap, rinsed well with tap water and then allowed to dry for 15-20
min, before application of the next dose of the test sample. The % protective
efficacy (PE) was calculated as follows:
Where PCM is the percent control mean and PTM is the percent test mean of mosquitoes landing on the control and treated arms respectively.
Antimosquito Tanzanian Plant Species: Various medicinal plants abound in Tanzania and other parts of the world possess insecticidal activity. Over 20 Tanzanian plant species have been established to be potential insecticidal agents against different species of mosquitoes. In the same line of investigations several mosquitocidal chemical compounds, some with novel chemical structures, have been isolated and characterized. The insecticides properties were categorized as larvicidal, growth inhibitor, adulticidal and repellent agents. Thus, from the late 1990s to the present several Tanzanian plant species have been investigated by various researchers for their anti-mosquito properties. These include, Neorautanenia mitis, known as Lidupala by Hehe tribe in Iringa region, where it is used as an insecticide for insect infestation in maize. Four mosquitocidal flavonoids with larvicidal and adulticidal activities, pachyrrhizine, neorautanone, neoduline, 4methoxyneoduline, neotenone and nepseudin were obtained from N. mitis (Joseph et al., 2004). Two asteranthines compounds with mosquitocidal activity 2',3'-epoxyasteranthine and 2',3'dihydroxyasteranthine were obtained from larvicidal root and stem barks of Asteranthe lutea. Compounds cleistenolide and monoterpene espintanol were established to be the mosquitocidal constituents of Cleistochlamys kirkii and Uvaria scheffleri, respectively (Nkunya et al., 2004; Samwel et al., 2007).
Phytochemical investigations of the dichloromethane and ethanol extracts of the cytotoxic root barks of Hugonia castaneifolia and H. busseana (Linaceae) yielded the rosane diterpenoids hugorosenone and 18hydroxyhugorosenone, and tetracosyl-(E)-ferrulate and a sesquiterpenoid 4-methoxy-5,9-oxahimachal-9ene, respectively that exhibited mosquito larvicidal activity against An. gambiae after 24 and 48 h exposure (Baraza et al 2007; Baraza et al., 2008).
Investigations of the three plant species of genera Tessmannia endemic to Tanzania yielded several mosquitocidal compounds, most of them with novel chemical structures. These include ent-clerodanoid compounds 18-oxocleroda-3,13(E)-dien-15-oic acid and ent-(18-hydroxy-carbonyl)-cleroda-3,13(E)-dien15-oate, 2-oxo-ent-cleroda-3,13(Z)-dien-15-oic acid and cis-2-oxo-ent-cleroda-13(Z)-en-15-oic acid, and O-(3-hydroxy-4-hydroxycarbonyl-5-pentylphenyl)-3chloro-4-methoxy-5-pentyl-2-oxybenzoic acid from Tessmannia martiniana var pauloi and T. martiniana var martiniana, respectively (Kihampa et al., 2010). Tessmannia densiflora yielded larvicidal compounds nor-halimanoid diterpenoids tessmannic acid and methyltessmannoate (Kihampa et al., 2009a). The antimosquitocidal phenylpropenoids Omethyleugenol, O-methylisoeugenol and 2,3dimethoxycinnamaldehyde were from the stem and root bark extracts of Uvariodendron pycnophyllum (Diels) R.E. Fr. The extracts and compounds exhibited activity with LC50 values in the range 17-59 ppm against the Anopheles gambiae s.s Giles mosquito larvae, while the constituent phenylpropenoids showed long term mortality effects to adult An. gambiae mosquito on impregnated bednets, and mosquito repellency that was stronger than the activity of the standard repellent DEET (Kihampa et al., 2010).
Mosquito larvicidal furanonaphthaquinones regioisomers (LC50 = 5.48–5.70
ppm in 72 h), the lantadene triterpenoid camaric acid (LC50 = 6.19 ppm in 72
h) and lupine triterpenoid betulinic acid (LC50 < 10 ppm in 72 h) were obtained
from Lantana viburnoides sp viburnoides var kisi (A. rich)
Verdc (Verbenaceae) (Innocent et al., 2008a). Investigations of polar
extract of Kotschya uguenensis Verdc (Fabaceae) showed very strong growth
disruption activity of larvae of Anopheles gambiae s.s (Diptera: Culicidae)
by forming elongated guts and resulting in eventual death (Innocent et al., 2008b).
Several other Tanzanian plant species have already been bio-prospected for mosquito larvicidal activity. Their crude extracts from leaves, stem and root barks, were established to have significant larvicidal activity against A. gambiae larvae under laboratory trials. Some of these plant species include Hoslundia opposita Vahl (Lamiaceae), Uvaria faulknerae Verdc., U. lungonyana Vollesen., U. kirkii Hook. F., U. leptocladon Oliv., Annona squamosa L., Asteranthe asterias (S.
Moore) Engl. & Diels., A. lutea Vollesen, Polyalthia tanganyikensis Vollesen, Lettowianthus stellatus Diels., Uvariodendron usambaranse Diels. (Annonaceae), Croton sylvaticus L (Euphorbiaceae) (Kihampa et al., 2009b).
Challenges of formulating mosquitocidal agents: Despite the successes
that have been achieved, several challenges have also been encountered in screening
extracts of many plant species. The bioactivity trends in plant extracts versus
the constituent natural products has been observed to be a subject of many phenomena.
The extracts activity may depend on the plant part used, test organism, method
and time of collection, method of extraction, solvent used and geographical location
of the plant. In this regard, proper screening methods are of paramount importance
in obtaining promising insecticides from plants. It is important to take the
seasonal variations of the constituents into consideration in order to maximize
the yield of such compounds at the time when they are metabolized in abundance.
Consideration must also be given to the fact that most active principles in plants
would show low stability, and as such they are usually lost during the harvesting,
drying, extraction and isolation processes. Again this factor needs to be recognised
when embarking onto bioprospecting ventures for bioactive compounds.
The high activity shown by some extracts conforms to the suggestion that plants never defend themselves against insects with a mono-component system.
Normally, for this purpose plants are said to use numerous constituent compounds, whether biosynthetically related or unrelated. Individual plant species are known to produce a variety of biosynthetically distinct metabolites and usually insects would perceive and react to these compounds as mixtures rather than as individual compounds. This could also be supported by some other evidence that natural mixtures do act synergistically. In some cases the activity of the crude extracts are less active than the pure compounds, such phenomenon could be explained by assuming that the activity of the pure compounds in the crude mixtures is masked by the other, less active or completely inactive minor constituents. These studies have therefore considered these findings as challenges which have to be clarified when considering use of a particular plant in crude form or as semi purified fractions.
In general, plant species of the same or closely related genera would contain the same or similar active principles. Consequently, a screening system which is successful with one species should also be attempted with closely related species. As such it proved useful to invoke the same separation techniques for compounds from each of the three Tessmannia species (Kihampa et al., 2010a; Kihampa et al., 2010b).
Several other major challenges have been realised that include the isolation of small amounts of pure compounds which are not sufficient to conduct bioassay tests. Likewise, limits in the availability of essential facilities hamper the ability to carry out spectroscopic experiments such as one-and twodimensional NMR spectroscopy, and MS and X-ray diffraction analysis for structural elucidation and stereochemical and conformational assignments, particularly of enantiomeric structures.
CONCLUSION
Chemicals derived from plants offer promise future mosquito control programs
as effective mosquito control agents, and biorational alternative to organic
synthetic pesticides. The fact that these chemicals are from natural sources,
with a high degree of biodegradation, makes them environmentally sound control
agents. Therefore, with an ever increasing public interest and awareness in
the environment, in both developed and developing countries, positive public
perception of natural insecticides is an added incentive for their development
and use. Thus, as the investigations in natural products research continue
to develop and proceed towards the discovery of novel natural insecticides
from botanical resource, future studies should aim at improving strategies
of evaluating extracts, re-collect, re-isolate and/or synthesize authentic
samples of natural product(s) and their derivatives to afford gram quantities
for follow-up bioassay screens so as to update the available chemical and biological
data. Simple blends and formulations of plants materials either in powder or
water extracts as used in local community should be scientifically developed,
this would be important to be used to the community where most of the people
can not afford the cost of modern insecticides.
REFERENCES
- Baraza, LD; Joseph, CC; Munissi, JJE; Nkunya, MHH; Arnold, N; Porzel, A; Wessjohann, L (2008). Antifungal rosane diterpenes and other constituents of Hugonia castaneifolia. Phytochem 69(1): 200-205.
- Baraza, LD; Joseph, CC; Nkunya, MHH (2007). “A new cytotoxic and
larvicidal himachalenoid, rosanoids and other constituents of Hugonia busseana.”Nat
Prod Res 21(11): 1027-1031.
- Becker, N; Petric, D; Zgomba, M; Boase, C; Dahl, C; Lane, J; Kaiser, A
(2003). Mosqutitoes and Their Control, Khuwer Academic/Plenum Publishers,
New York, p 382.
- Curtis, CF; Lines, JD; Lu, B; Renz, A (1991). Natural and synthetic repellents.
In: control of disease vector in the community. Curtis, C.F. (Ed.). Wolfe
Publishing Ltd., London, p 75-92.
- Dev, S; Koul, O (1997). Insecticides of Natural Origin, Hartwood Academic
Publishers, India.
- Farnsworth, NR (1966). Biological and phytochemical screening of plants.
J Pharmacol Sci 55: 225-276.
- Innocent, E; Joseph, CC; Gikonyo, NK; Moshi, MJ; Nkunya, MHH; Hassanali,
A (2008a). Mosquito larvicidal constituents from Lantana viburnoides sp viburnoides var kisi (A.
rich) Verdc (Verbenaceae). J Vector Borne Disease 45: 240–244.
- Innocent, E; Joseph, CC; Gikonyo, NK; Nkunya, MHH; Hassanali, A (2008b).
Growth disruption activity of polar extracts from Kotschya uguenensis (Fabaceae)
against Anopheles gambiae s.s.(Diptera: Culicidae) larvae. Interna
J Trop Insect Sci 28: 220–224.
- Joseph, CC; Ndoile, MM; Malima, RC; Nkunya, MHH (2004). “Larvicidal
and mosquitocidal extracts, a coumarin, isoflavonoids and pterocarpans from Neorautanenia mitis.”Trans
Royal Soc Trop Med Hyg 98: 451-455.
- Kakkilaya, BS (2007). History of Malaria Control, Malaria Site, http://www.malariasite.com/malaria/history_control.htm.
- Khafagi, IK; Dewedar, A (2000). The efficiency of random versus ethno-directed
research in the evaluation of Sinai medicinal plants for bioactive compounds.
J Ethnopharmacol 71: 365-376.
- Kihampa, C; Nkunya, MHH; Joseph, CC; Magesa, S; Hassanali, A; Hydenreich,
M; Kleinpeter, E (2009a). Mosquitocidal and Antimicrobial Nor–halimane
Diterpenes From Tessmannia densiflora. Phytochem 70: 1233-1238.
- Kihampa, C; Joseph, CC; Nkunya, MHH; Magesa, MS; Hassanali, A; Hydenreich,
M; Kleinpeter, E (2009b). Larvicidal and IGR activity of extract of Tanzanian
plants against malaria vector mosquitoes. J Vector Borne Diseases 46: 145-152.
- Kihampa, C; Nkunya, MHH; Joseph, CC; Magesa, S;
Hassanali, A; Hydenreich, M; Kleinpeter, E
(2010a). Antimosquito and antimicrobial
clerodanoids and a chlorobenzenoid from
Tanzanian Tessmannia species. Nat Prod
Commun 5(2): 175-178.
- Kihampa, C; Nkunya, MHH; Joseph, CC; Magesa, SM (2010b). Antimosquito phenylpropenoids
from Uvariodendron pycnophyllum (Diels) R.E.Fr. J Appl Sci Environ
Manage 14(3): 29-32.
- Meyer, BN; Ferrigin, NR; Putnam, JE; Jacobson, LB; Nicholas, DE; McLaughling,
JL (1982). Brine shrimp: A convenient general bioassay for active plant constituents.
Planta medica 45: 31-34.
- MOH (Ministry of Health, 2006). “The United Republic of Tanzania, Guidelines for the management of malaria for health service providers.”Malaria
Control Series 12, p iii, 2.
- Nkunya, MHH (2004). Chemistry for Disease and Poverty Eradication in Africa,
Proceedings of the 9th International Chemistry Conference in
Africa (ICCA-9), Arusha, Tanzania, p 1.
- Nkunya, MHH; Jonker, SA; de Gelder, R; Wachira, SW; Kihampa, C (2004). “Dimeric monoterpenoid (±)-schefflone:
A trimeric monoterpenoid from the root bark of Uvaria scheffleri.”Phytochem
65: 399-404.
- Sachs, J; Malaney, P (2002). “The economic and social burden of malaria.”Nature
415: 680-685.
- Samwel, S; Mdachi, SJM; Nkunya, MHH; Irungu, BN; Moshi, MJ; Moulton, B;
Luisi, BS (2007). Cleistenolide and Cleistodienol: Novel bioactive constituents
of Cleistochlamys kirkii. Nat Prod Commun 2(7): 737-741.
- The Wellcome Trust. (1999). Research directions in Malaria, Wellcomes News
Supplements, volume 6.
- Yamaguchi I. (1990). Recent development in the field of pesticides and
their application to pest control. Proceedings of the international seminar,
Shenyang, China. p. 164.
- Yekutiel P. (1980). Eradication of Infectious Diseases: A Critical Study.
University of Califonia, p 39.
- Sukamar, K; Perich, MJ; Boobar, LR (1991). “Botanical derivatives
in mosquito control: A review.”J Am Mosq Contr Ass 7: 210-237.
Copyright 2011- Journal of Applied Sciences and Environmental Management
|