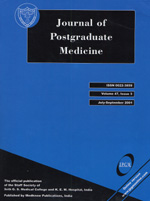
|
Journal of Postgraduate Medicine
Medknow Publications and Staff Society of Seth GS Medical College and KEM Hospital, Mumbai, India
ISSN: 0022-3859 EISSN: 0972-2823
Vol. 49, Num. 3, 2003, pp. 236-245
|
Journal of Postgraduate Medicine, Vol. 49, No. 3,
July-Sept, 2003, pp. 236-245
Symposium: Diet in Primary Prevention of Disease
Can We Prevent Parkinson's and Alzheimer's Disease?
Kedar NP
Center for Vitamins and Cancer Research, Department
of Radiology, School of Medicine,
Correspondence Address: Campus Box C-278, UCHSC,
4200 E. 9th Ave., Denver, Colorado, 80262 Kedar.Prasad@UCHSC.edu
Code Number: jp03065
ABSTRACT
Parkinson's disease (PD) and Alzheimer's (AD) are major progressive neurological
disorders, the risk of which increases with advancing age (65 years and over).
In familial cases, however, early onset of disease (about 35 years) is observed.
In spite of extensive basic and clinical research on PD and AD, no preventive
or long-term effective treatment strategies are available. Several studies
have indicated that oxidative stress is a major risk factor for the initiation
and progression of sporadic PD and AD. Even α-synuclein and β-amyloid fragments
that are associated with the PD and AD, respectively, mediate part of their
action via oxidative stress. Therefore, reducing oxidative stress appears to
be a rational choice for the prevention and reduction in the rate of progression
of these neurological disorders. This review provides a brief description of
the epidemiology and pathogenesis of PD and AD, and the scientific rationale
for the use of multiple antioxidants in the prevention of these neurological
diseases.
Keywords: Parkinson's disease, Alzheimer's disease, Antioxidants,
Oxidative stress
Parkinson's disease (PD) and Alzheimer's (AD) are major progressive neurological
disorders, the risk of which increase with advancing age (65 years and over).
Only about 5% of these diseases are due to hereditary factors; the remaining
cases are considered to be idiopathic or sporadic. In spite of extensive basic
and clinical studies on PD and AD, no preventive or long-term effective treatment
strategies are available. The analysis of existing data suggests that increased
oxidative stress is a major factor for the initiation and progression of these
neurological diseases.[1],[2] We
have proposed that oxidative and nitrosylative stress caused by reactive oxygen
species (ROS), such as O,-. OH., R, RO2., and H2O2, and reactive nitrogen species
(RNS), such as NO., NO2., are major intermediate risk factors for a diverse
group of neurotoxins that could initiate and promote neurodegeneration in the
brains
of PD[3] and AD[4] patients.
Therefore any strategy that can reduce the levels of oxidative and nitrosylative
stress beginning from a young age may reduce the risk of these neurological
diseases during old age. We have further proposed that epigenetic components
of neurons
(mitochondria, other organelles, membranes, protein modifications) rather than
nuclear genes may be the primary targets for the action of neurotoxins, including
free radicals in the development of PD and AD.[3],[4] Therefore,
improving the functions of these epigenetic components of neurons, and protecting
them from further damage by free radicals in patients with early phase disease
may help to reduce the rate of progression of symptoms in both PD and AD.
The purpose of this review is to discuss recent advances made in the understanding
of the aetiology and pathogenesis of PD and AD. It also proposes a scientific
rationale for the use of multiple antioxidants at appropriate doses, dose schedules
and type of antioxidants to reduce the risk of these diseases in high risk
populations and the progression of disease in patients with an early phase
disease when no
treatment is given.
EPIDEMIOLOGIC STUDIES
Results from human epidemiologic studies [3],[4] showed
that exposure to excessive amounts of manganese such as observed among manganese
miners increases the incidence of a PD-like disease. Increased incidence of
PD-like disease is also seen among users of the designer drug, meperidene,
which contains 1-methyl-4-phenyl 1,2,3,6
tetrahydropyridine (MPTP), a neurotoxic byproduct formed during the synthesis
of this drug. The harmful effects of MPTP and excessive exposure to Mn on DA
neurons may in part be mediated through free radicals. Although no particular
dietary risk factors for PD have been found, the consumption of nuts, and salad
oil (pressed from seeds) have been found to be of protective value. A large
community-based study in the Netherlands has reported that vitamin E consumption
was significantly lower among patients with PD than among controls.
Epidemiologic investigations of AD have also failed to identify environmental,
dietary and life-style-related risk factors that could be used for the prevention
of this disease. Chronic inflammatory reaction, however, appears to be a major
risk factor. This hypothesis is supported by the epidemiologic studies that
showed that rheumatoid arthritis patients, who were on high doses of NSAIDs,
had a reduced incidence of AD. Accumulation of increased levels of Al and iron,
Cu or Zn appears to be also associated with increased risk of AD. Depression
symptoms are associated with the increased risk of AD.[5] Contrary
to the results published on European and Asian populations, women were not
at increased risk for AD.[6]
PATHOGENESIS OF PD AND AD
Parkinson's disease is characterized by the loss of dopaminergic neurons in
the pars compacta of the substantia nigra and by intraneuronal cytoplasmic
inclusions called Lewy bodies.[7],[8] The
diagnosis of AD is made by postmortem analysis of brains of patients with dementia.
The presence of intracellular neurofibrillary tangles (NFT) containing hyperphosphorylated
tau protein and apolipoprotein E, and extracellular senile (neuritic) plaques
containing many proteins, including non-soluble b-amyloid, a-synuclein, ubiquitin,
apolipoprotein E, presenilins, and alpha-antichymotrypsin, are considered hallmarks
of AD.[9],[10] PD
and AD generally are associated with the increased cerebral accumulation of
α-synuclein and Aβ, respectively. However, some patients have clinical and
pathological features of both diseases, suggesting that both α-synuclein and
APP may be involved in these diseases.[11]
SOURCES OF OXIDATIVE
STRESS IN NORMAL BRAIN
The brain utilizes about 25% of respired oxygen even though it represents
only 5% of the body weight. Free radicals are generated during the normal intake
of oxygen, during infection, and during normal oxidative metabolism of certain
substrates. During normal aerobic respiration, the mitochondria of one rat
nerve cell will process about 10 [12] oxygen
molecules and reduce them to water. During this process, superoxide anion,
hydrogen peroxide and hydroxyl are produced. In addition, partially reduced
oxygen, which represents about 2% of consumed oxygen, leaks out from the mitochondria
and generates about 20 billion molecules of O2-. and H2O2 per cell per day.[12] During
bacterial or viral infection, phagocytic cells generate high levels of nitric
oxide (NO), O2-., and H2O2 in order to kill infective agents; however, these
radicals can also damage normal cells.[12] During
degradation of fatty acids and other molecules by peroxisomes, H2O2 is produced
as a byproduct. During oxidative metabolism of ingested toxins, free radicals
are also generated.
Some brain enzymes such as monoamine oxidase (MAO), tyrosine hydroxylase, and
L-amino acid oxidase produce H2O2 as a normal byproduct of their activity.
Furthermore, auto-oxidation of ascorbate and catecholamines generates H2O2.
Oxidative stress can also be generated by Ca2+-mediated activation of glutamate
receptors. The Ca2+-dependent activation of phospholipase A2 by N-methyl-D-aspartate
(NMDA) releases arachidonic acid, which then liberates O2-. during the biosynthesis
of eicosanoid.[13] Another radical, NO, is
formed by nitric oxide synthase stimulated by Ca2+. NO can react with O2-. to
form peroxynitrite anions that can form OH., the highly reactive hydroxyl radical.
Some enzymes such as xanthine oxidase and flavoprotein oxidase (e.g. aldehyde
oxidase) also form superoxide anions during metabolism of their respective
substrates. Oxidation of hydroquinone and thiol, and synthesis of uric acid
from purines form superoxide anions. Several different types of radicals are
constantly formed in the brain. Their levels can be increased by enhanced turnover
of catecholamines, increased levels of free iron, impaired mitochondrial function,
decreased glutathione levels, decreased levels of catalase, glutathione peroxidase
or superoxide dismutase. Cigarette smoking increases the level of NO by about
1000 ppm and depletes antioxidant levels.[14],[15] Dietary
phenolic compounds such as chlorogenic and caffeic acid when oxidized act as
free radicals.
These studies suggest that the brain generates high levels of ROS and RNS every
day. Paradoxically, the brain is least prepared to handle this excessive load
of free radicals. It has low levels of both antioxidant enzyme systems and
dietary antioxidants. These inherent biological features make the brain very
vulnerable to oxidative and nitrosylative stress. Despite this, the risk of
idiopathic PD or AD becomes significant only after the age of 65 or more. This
is due to the fact that neurons exhibit a high degree of plasticity in maintaining
normal brain functions. The fact that clinical symptoms PD and AD appear only
when a significant number of neurons are lost, supports the value of plasticity
of the neurons in maintaining normal brain function. Supplementation with antioxidants
vitamin A, C, and E, natural β-carotene, co-enzyme Q10, α-lipoic acid, N-acetyl-cysteine
and NADH may reduce the rate of loss of neurons.
EVIDENCE
FOR THE INVOLVEMENT OF OXIDATIVE AND NITROSYLATIVE STRESS IN IDIOPATHIC
PD
BRAIN
The normal brain has the highest concentration of unsaturated fatty acids
compared to other organs and these fatty acids are very susceptible to lipid
peroxidation.[16] The substantia nigra (SN)
is the primary area of the brain that undergoes degeneration in PD. Autopsy
samples of SN from PD brains revealed increased oxidant levels and decreased
antioxidant levels. For example, increased levels of free iron were demonstrated
in autopsy samples[17] as well as in brains
of living PD patients by iron-mediated contrast magnetic resonance imaging
(MRI).[18] SN samples from PD brains have
reduced levels of antioxidant enzymes,[19],[20] and
reduced levels of antioxidants.[21] The above
changes increase the balance in favor of a pro-oxidant environment, which can
increase oxidative damage. Indeed, evidence of oxidative damage in the autopsy
samples of brain of PD has been observed.[22],[23],[24]
EVIDENCE
FOR THE INVOLVEMENT OF OXIDATIVE STRESS IN FAMILIAL PD BRAIN
Point mutations in the a-synuclein gene which codes for a presynaptic nerve
terminal protein are associated with familial PD.[25] Immortalized
DA neurons expressing mutated a-synuclein gene showed increased sensitivity
to 6-hydroxydopamine.[26] In addition, it
has been reported that a-synuclein-induced apoptosis may be due to increased
oxidative damage.[27],[28],[29] In
another study, it has been shown that in some cases nitrated a-synuclein is
present in inclusion bodies of AD and PD.[30] This
suggests that a-synuclein increases oxidative damage and nitrosylative damage
that may also play a crucial role in neurodegeneration. A study has reported
that mutations in the Parkin gene are associated with autosomal recessive juvenile
parkinsonism (AR-JP).[31] It is unknown whether
the mutated Parkin gene mediates its effect on neurons via increasing oxidative
stress.
EVIDENCE
FOR THE INVOLVEMENT OF OXIDATIVE AND NITROSYLATIVE STRESS IN IDIOPATHIC
AD
BRAIN
Increased oxidative stress has been implicated in the loss of neurons associated
with AD.[32] Mitochondria may be one of the
most sensitive primary targets that increase oxidative stress in adult neurons.[33],[34] This
may be due to the fact that mitochondrial DNA (mtDNA) does not encode for any
repair enzymes, and, unlike nuclear DNA, it is not shielded by protective histones.
In addition, mtDNA is in close proximity to the site where free radicals are
generated during oxidative phosphorylation. Indeed, an increased frequency
of mutations in mtDNA has been found in autopsy samples of AD brains,[33] and
thus mitochondrial defects may be involved in the pathogenesis of AD.[34],[35] A
defect in energy production may also increase the sensitivity of neurons to
excitatory amino acids.[36] Impaired mitochondria
increased generation of potentially amyloidogenic derivatives.[37] Excess
of free Zn is found in the autopsied brain of AD and increased free Zn can
impair mitochondrial function.[38] It is interesting
to note that in AD patients who carry ApoE4 allele of ApoE gene, the clinical
Dementia Rating (CDR) correlated better with KGDHC activity than with densities
of neuritic plaques and NTFs; however, in patients without ApoE4, the CDR correlated
better with plaques and NTFs than with KGDHC activity.[39] This
suggests that mitochondrial dysfunction may be more important for the development
of AD in patients who carry ApoE4 allele than in those who do not.
Increased oxidative stress may enhance intracellular accumulation of Ab in
neurons.[40] In addition, studies show that
membrane containing oxidatively damaged phospholipids accumulated Ab faster
than membrane containing only normal saturated phospholipids.[41] It
has been proposed that one of the mechanisms of action in Ab neurotoxicity
is mediated by free radicals.[42] This was
confirmed by a series of studies on substitutions of amino acid,[43] and
also by the fact that vitamin E protects neuronal cells in culture against
Ab-induced toxicity.[44] Experiments on a
transgenic mouse model of AD support the concept that Ab-induced neurotoxicity
is mediated by oxidative stress. For example, it has been reported that Cu/Zn
superoxide dismutase (SOD), and hemoxygenase-1 (HO-1), markers of oxidative
stress, were elevated in aged transgenic mice.[45]
Other evidence of increased oxidative stress in AD include the following: (a)
the serum levels of vitamins A, E and b-carotene were lower in patients with
AD (who were well nourished) than in control patients;[46] (b)
higher expression of heme oxygenase is found in the autopsy samples of brains
of AD patients;[47] (c) increased consumption
of oxygen is found in AD patients;[48] (d)
increased activity of glucose-6-phosphate dehydrogenase is found in the autopsy
samples of AD brain;[49] and (e) activation
of calcium-dependent neural proteinase (calpain) is found in the autopsy samples
of AD brains which may trigger events leading to the formation of free radicals;[50] (f)
homogenates of frontal cortex from the autopsy samples of AD brains revealed
a 22% higher production of free radicals and, in the presence of iron, a 50%
higher production of free radicals than those of age-matched normal controls;[51] (g)
besides oxidative stress, nitrosylative stress, which is primarily mediated
by peroxynitrites, can potentially exacerbate the pathogenesis of AD;[52] (h)
increased neuronal nitric oxide synthase (nNOS) expression in reactive astrocytes
correlated with apoptosis in hippocampal neurons of AD brains;[53] (i)
glutamine synthetase, a highly sensitive enzyme to oxidative stress, showed
decreased activity in the autopsy samples of AD brains;[54] (j)
the level of glutathione transferase is decreased in ventricular CSF and in
the autopsy samples of AD brains compared to brains from age- matched controls;[55] and
(f) increased levels of oxidized proteins are found in the blood of both AD
patients and their relatives when compared with non-AD control.[54] Evidence
for oxidative and nitrosylative damage at autopsy in the brains of AD patients
have also been reported.[55],[56]
EVIDENCE
FOR THE INVOLVEMENT OF OXIDATIVE STRESS IN FAMILIAL AD BRAIN
In some familial AD, mutations (about seven) in the APP gene have been reported,
all of which increase the production of b-amyloid;[57] however,
this accounts for less than 1 % of all familial AD. Mutations (about fifty)
in presenilin-I gene have been found in about 50% of familial AD,[57] whereas
mutations in presenilin-II have been observed in less than 1% of familial AD.[58] The
interaction between APP and presenilin I or presenilin II may increase production
and release of b-amyloid.[59] It should be
noted that in spite of mutations in APP and presenilin genes, a minimum of
about 30 years is needed for the development of familial AD. This suggests
that the products of mutated genes by themselves are not toxic. It is possible
that cells expressing these gene mutations may become more sensitive to neurotoxins
including oxidative and nitrosylative stress. Indeed, we have shown that the
expression of high levels of wild type APP in differentiated neuroblastoma
cells makes these cells more sensitive to neurotoxins such as PGE2, PGA1, oxidative
and nitrosylative stress.[60]
INVOLVEMENT
OF NUCLEAR GENES IN IDIOPATHIC PD
No genetic defects in idiopathic PD have been demonstrated as yet. Polymorphism
in certain genes such as those that code for dopamine-transporter protein,[61] alpha-1-antichymotrypsin,[62] monoamine
oxidase B[63] and cytochrome P4501A1 (CYP1A1)[64] have
been associated with increased risk of idiopathic PD. Polymorphism in DA neurons
could lead to increased accumulation of neurotoxins in these cells. Since polymorphism
in these genes was measured in peripheral cells, it is difficult to suggest
that it also occurs in DA neurons. In addition, there is no direct evidence
that polymorphic genes are either neurotoxic or increase the sensitivity of
DA neurons to neurotoxic agents.
INVOLVEMENT
OF
NUCLEAR GENES IN IDIOPATHIC AD
There is no solid evidence for nuclear gene defects that can increase the
risk of idiopathic AD, although varying degrees of association between certain
gene defects and onset of this disease exist. Several studies have suggested
that persons who are homozygous for the apolipoprotein E (APOE), e4 allele,
develop AD 10-20 years earlier than those who have e2 or e3 alleles.[65] Even
persons who are heterozygous for e4 allele develop AD 5-10 years earlier than
those who have e2 or e3 alleles.[66] About
40 % of idiopathic AD is associated with the presence of e4 allele, and it
is present in the senile plaque.[66] These
data suggest that the presence of e4 allele could be an important risk factor
for AD. However, it was shown that this allele is neither essential nor specific
for the development of AD.[66] Thus, the role
of this APOE allele in neurodegeneration is uncertain. Mutation in the ubiquitin
gene[67] and down-regulation of presenilin
II[68],[69] have
been observed in the autopsy samples of AD brains.
EPIGENETIC
COMPONENTS OF NEURONS ARE PRIMARY TARGETS OF ACTIONS OF A DIVERSE GROUP
OF NEUROTOXINS IN IDIOPATHIC PD AND AD
We have proposed a hypothesis that epigenetic components (mitochondria, proteasomes,
post-translational modification of proteins) rather than nuclear genes are
the primary targets for the action of diverse groups of neurotoxins in idiopathic
PD and AD.[3],[4] For
example, mitochondrial dysfunction is associated with both PD and AD; therefore;
it may represent an early event in the pathogenesis of these neurological disorders.[3],[4]
Even in cases of familial AD, the products of mutated genes by themselves are
not neurotoxic, rather they affect epigenetic components such as post-translational
modification of proteins (increased processing of APP to Aβ40 and Aβ42) that
could increase oxidative stress and/or make neurons more sensitive to oxidative
stress. We also suggest that the shift in processing of APP to Aβ42 in AD brain
is an example of post-translational modification of protein caused by certain
neurotoxins.
Proteasome represents another example of an epigenetic component and it regulates
certain transcriptional factors by splicing inactive peptide fragments on to
active ones, and protein levels by degrading ubiquitin-conjugated abnormal
proteins. Therefore, inhibition of proteasome in neurons can initiate and promote
neurodegeneration. Indeed, the role of proteasome inhibition has been proposed
for the degeneration of neurons in AD brains,[70],[71] and
Ab is one of the factors that inhibits proteasome activity.[72] A
defect in ubiquitin conjugate enzymes[73] or
a mutation in ubiquitin (Ub) could also
impair removal of unwanted proteins via proteasome. We have shown that inhibition
of proteasome by lactacystin causes rapid degeneration of cAMP-induced differentiated
neuroblastoma cells in culture.[74] Furthermore,
we have suggested[4] that increased accumulation
of ubiquitin[10],[75] and
hyperphosphorylated tau protein[76] in AD
brains is a reflection of inhibition of proteasome activity.
Cholesterol levels also represent one of the epigenetic components of neurons.
Epidemiologic studies showed that hypercholesterolemia may be a risk factor
in the development of AD,[77],[78],[79],[80] and
that lovastatin, an inhibitor of HMG CoA reductase, reduces the risk of AD
in hypercholesterolemic patients.[81] The
role of cholesterol was confirmed in the transgenic animal model of AD in which
high dietary cholesterol increases Ab accumulation and thereby accelerates
AD-related pathology in animals.[82] An accumulation
of Ab can be reversed by removing cholesterol from the rabbit's diet.[82] Inhibitors
of HMG CoA reductase decrease the production of Ab in rabbit and in fetal rat
hippocampal neurons in culture.[83] These
results suggest that some of the effects of cholesterol are primarily mediated
via Ab rather than via poor circulation due to thickening of the arteries.
Statins with a closed- ring structure (lovastatin, simvastatin, mevastatin)
are metabolized in vivo to an open-ring structure which then inhibits HMG CoA
reductase activity. Recently, we have demonstrated that mevastatin with a closed-ring
structure caused rapid degeneration of differentiated neuroblastoma (NB) cells
in culture, whereas, pravastatin with an open-ring structure did not.[84] Mevastatin-induced
degeneration of differentiated NB cells may be related to inhibition of proteasome
activity.[84] These studies suggest that lowering
cholesterol levels could reduce the risk of AD, whereas the presence of increased
amounts of unmetabolized statins with a closed -ring structure could increase
the risk of AD. Another study has shown that cholesterol-fed rabbits placed
on distilled water have a 28% reduction in the level of b-amyloid in comparison
to those on tap water.[85]
LABORATORY STUDIES
WITH ANTIOXIDANTS IN PD
In order to investigate the effect of antioxidants in an animal model of Parkinsonism,
it is essential to study whether antioxidant supplementation can increase brain
levels of antioxidants. It has been reported that dietary supplementation with
dl-alpha tocopherol (1000 I.U./day) for 4 months increased rat brain levels
of vitamin E by about 1.4 fold.[86] The brain
and cerebrospinal fluid levels of vitamin E also increased by 2-fold in dogs
treated with vitamin E supplements for 2 years.[87] These
studies suggested that supplementation with vitamin E could be of protective
value in an animal model of PD. Indeed, supplementation with vitamin E and
vitamin C88 protected rats against 6-hydroxydopamine-induced striatal damage
(rat model of PD). In vitro experiments reveal that vitamin E protects neurons
against 6-hydroxydopamine which is known to mediate its action in part by free
radicals.[89] Additional studies on the efficacy
of multiple antioxidants in reducing the symptoms of PD in animal models are
needed.
LABORATORY STUDIES
WITH ANTIOXIDANTS IN AD
The effect of antioxidants in animal model of AD has not been investigated.
In vitro studies show that vitamin E can protect neurons against neurotoxins
such as glutamate[90] which is known to mediate
its action in part by free radicals. Vitamin E also protects rats against aggregated
Ab-induced behavioral impairments.[91] Lysosomes
play a key role in preventing the formation of amyloid deposits and senile
plaques, and vitamin C improves lysosomal functions of human brain astrocytes[92] and
thereby preserves cellular function. Additional studies on the efficacy of
multiple antioxidants in reducing the symptoms of AD in animal models are needed.
CLINICAL STUDIES
WITH
ANTIOXIDANTS IN PD
In a preliminary study, supplementation with vitamin E (3,000 I.U./day) and
vitamin C (3,000 mg/day) increased the time interval for requiring L-dopa therapy
by about 2-4 years in 75% of patients when compared to historical controls;
however, 16% of patients on vitamin therapy did not require L-dopa therapy
at the time of writing the manuscript (about 8 years).[93] In
contrast to the above study, a large clinical, double-blind, and placebo-controlled
study involving 800 patients in the early stages of untreated PD was initiated
to evaluate the efficacy of alpha-tocopherol and deprenyl on the rate of progression
of PD.[94] This study was referred to as Deprenyl
and Tocopherol Antioxidant Therapy of Parkinsonism (DATATOP). The major endpoint
of this study was the time interval between initiation of experimental treatment
and need for L-dopa treatment. Synthetic dl-alpha-tocopherol at a daily dose
of 2,000 I.U. was given orally. This study failed to show any significant improvements
based on the proposed endpoint.[94] This clinical
study failed to consider the consequence of metabolism of high dose vitamin
E, multifactorial nature of the disease, reduced levels of other antioxidants
such as glutathione, and the value of NADH that increases the level of dopamine.
CLINICAL STUDIES
WITH
ANTIOXIDANTS IN AD
A controlled clinical trial with dl-α tocopherol (synthetic form; 2,000 IU/day)
in patients with moderately severe impairment from AD showed some beneficial
effects with respect to rate of deterioration of cognitive function[95] Although
this important clinical study supports the role of free radicals in the progression
of AD, the use of a single antioxidant, vitamin E, and the administration regime
(once a day) may not have been optimal for quenching all the various types
of free radicals that are produced in the brain. For example, It has been reported
that rat organs preferentially absorb the natural form of vitamin E;[96] therefore,
the use of synthetic vitamin E in any clinical study may not be useful. In
addition, the a-tocopherol form of vitamin E may not cross the blood-brain
barrier as efficiently as d-a-tocopheryl succinate (a-TS), since a-TS is more
soluble in ethanol, and enters the mammalian cell more readily than a-tocopherol.[97] Therefore
the use of a-TS may be beneficial. In addition, the use of a single antioxidant
may not be prudent for long-term therapy, because very high doses of a single
antioxidant that may be needed to produce a beneficial effect in AD patients,
and such high doses can cause a clotting defect.
In the same trial as above, selegiline (10 mg a day), a monoamine oxidase inhibitor,
or dl-a tocopherol slowed the progression of disease in patients with moderately
severe impairments from AD.[95] It was interesting
to note that there was no significant difference in the effect between the
groups receiving a combination of dl-a-alpha tocopherol and selegiline and
those receiving treatment with the individual agents.[95] In
our opinion, this was expected because both selegiline and vitamin E reduce
the levels of ROS, although by different mechanisms. For example, vitamin E
protects neurons by destroying formed free radicals ("quenching"), whereas
selegiline protects neurons by preventing the formation of ROS through inhibiting
oxidative metabolism of catecholamines.
PREVENTION STRATEGIES
FOR
PD AND AD
Prevention strategies can be developed if the risk and protective factors
for PD and AD are known. Based on current knowledge of risk and protective
factors, we propose prevention strategies for those who have no clinical symptoms
of PD or AD, and those who are at high risk for developing these neurological
disorders. This involves a diet rich in antioxidants and moderate supplements
with multiple antioxidants right from childhood that can maintain brain antioxidants
at levels that are higher than normally provided by the nature.
RATIONALE
FOR USING MULTIPLE ANTIOXIDANTS FOR PD AND AD PREVENTION
The biological rationale for using multiple antioxidants is described below.
Beta-carotene (BC) is more effective in quenching oxygen radicals than most
other antioxidants.[98] BC can produce certain
effects that cannot be produced by its metabolite vitamin A, and vice versa.[99] The
gradient of atmospheric (oxygen) pressure varies within the tissue. Some
antioxidants such as vitamin E are more effective quenchers of free radicals
in reduced oxygen pressure, whereas BC and vitamin A are more effective at
higher atmospheric pressure.[100] Vitamin
C is necessary to protect cellular components in aqueous environments, whereas
carotenoids, vitamins A and E protect cellular components in non-aqueous
environments. In addition, vitamin C is necessary for the activity of tyrosine
hydroxylase, which is the rate-limiting enzyme in the synthesis of catecholamines.
Vitamin C also plays an important role in maintaining cellular levels of
vitamin E by recycling the vitamin E radical.[101] Also,
oxidative damage produced by vitamin C (oxidized adenine nucleotides) could
be protected by vitamin E. We have reported that oral ingestion of a-TS (800
I.U./day) in humans increased plasma levels of not only a-tocopherol, but
also a-TS, suggesting that a-TS can be absorbed from the intestinal tract
before hydrolysis to a-tocopherol.[97] Levels
of reduced glutathione decrease in PD.[102] Glutathione
is effective in catabolizing H2O2 and anions. However, oral supplementation
with glutathione failed to significantly increase plasma levels of glutathione
in human subjects[103] suggesting that
this tripeptide is completely hydrolyzed in the G.I. tract. N-acetylcysteine
and a-lipoic acid that increase glutathione levels would be useful. Since
mitochondrial dysfunction is associated with PD, and since coenzyme Q10 and
nicotinamide adenine dinucleotide (reduced form, NADH) are needed for generation
of ATP by mitochondria, it is essential to use these antioxidants among the
high-risk populations. A study has shown[104] that
ubiquinol (coenzyme Q10) scavenges peroxy radicals faster than a-tocopherol,
but it is rapidly oxidized to give hydroperoxy radicals and/or superoxide.
Therefore, it is a weaker antioxidant than a-tocopherol. However, ubiquinol,
like vitamin C, can regenerate vitamin E in a redox cycle.[105] Coenzyme
Q10 administration improves clinical symptoms in patients with mitochondrial
encephalomyopathies.[106] NADH administration
(1.4 mg/Kg) has been useful in 415 PD patients.[107] In
addition to acting as an antioxidant, it can stimulate the production of
L-dopa in vivo[108] and dopamine in PC-12
cells, a dopaminergic cell line as well as ATP.[109] Selenium
is a co-factor of glutathione peroxidase; therefore, selenium supplementation
is essential.
RECOMMENDED
MULTIPLE ANTIOXIDANTS FOR PREVENTION
The levels of risk and protective factors in the brain may very from one individual
to another, depending upon the age, exposure to neurotoxins including free
radicals, pharmacokinetics and consumption of diets or supplements rich in
antioxidants. Therefore, we have divided recommendations based on age and population
at risk for PD and AD. These recommendations have been summarized in [Table-1].
RECOMMENDED
MULTIPLE ANTIOXIDANTS FOR EARLY PHASE PD
Early stage PD is referred to as a condition where no L-dopa therapy is required.
Therefore, any significant extension of this time interval would be an important
contribution to the management of PD. Recommended antioxidants have been summarized
in [Table-2].
RECOMMENDED
MULTIPLE ANTIOXIDANTS IN EARLY PHASE AD PATIENTS
Reactive oxygen species and reactive nitrogen species play an important role
in the progression of neurodegeneration in AD. Therefore, multiple antioxidant
supplements as an adjunct to standard therapy in the treatment of AD would
be more useful than the individual agents alone. NADH administration (10 mg/day
before meal) has been beneficial in a pilot study of 17 AD patients.[110] Selenium
is a co-factor of glutathione peroxidase, and Se-glutathione peroxidase also
acts as an antioxidant. Therefore, selenium supplementation together with other
antioxidants is also essential.
In addition to antioxidants, vitamin B-12 may have some role in the treatment
of AD. In most studies the serum levels of vitamin B-12 in AD patients were
significantly lower than controls, and this may partly contribute to degeneration
of neurons.[111] Indeed, vitamin B-12 supplementation
increased choline acetyltransferase activity in cholinergic neurons in cats[112] and
improved cognitive functions in AD patients.[113] Therefore,
the inclusion of vitamin B-12 in multiple antioxidant preparations may be useful.
Multiple antioxidants recommended for early phase AD patients are described [Table-2].
DIET AND LIFESTYLE RECOMMENDATIONS
Even though, there is no direct link between the diet and lifestyle related
factors and the risk of PD and AD or progression of these diseases, it is always
useful to include a balanced diet that contains low fat and plenty of fruits
and vegetables rich in antioxidants. Among fruits, blueberries and raspberries
are particularly important because of their protective role against oxidative
injuries in brain. Lifestyle recommendations include daily moderate exercise,
reduced stress and no tobacco smoking, avoiding exposure to pesticides, and
avoiding the intake of iron, Cu, Mn and Zn through supplements. These recommendations
are not specific to PD or AD. They are for general optimal health.
ACKNOWLEDGEMENTS
This study was supported by the US Public Health Service Grant AG 18285
REFERENCES
1. |
Olanow CW, Youdim MB. Neurodegeneration and neuroprotection in Parkinson's
disease. New York: Academic Press; 1996. Back to cited text no. 1 |
2. |
Schapira AH. Pathogenesis of Parkinson's disease. Baillieres Clin Neurol
1997;6:15-36 Back to cited text no. 2 [PUBMED] |
3. |
Prasad KN, Cole WC, Kumar B. Multiple antioxidants in the prevention
and treatment of Parkinson's disease. J Am Coll Nutr 1999;18:413-23 Back to cited text no. 3 [PUBMED] [FULLTEXT] |
4. |
Prasad KN, Cole WC, Prasad KC. Risk factors for Alzheimer's disease:
role of multiple antioxidants, non-steroidal anti-inflammatory and cholinergic
agents alone or in combination in prevention and treatment. J Am Coll Nutr
2002;21:506-22 Back to cited text no. 4 [PUBMED] [FULLTEXT] |
5. |
Green RC, Cupples LA, Kurz A, Auerbach S, Go R, Sadovnick D, et al.
Depression as a risk factor for Alzheimer disease. Arch Neurol 2003;60:753-9 Back to cited text no. 5 [PUBMED] [FULLTEXT] |
6. |
Edland SD, Rocca WA, Petersen RC, Cha RH, Kokmen E. Dementia and Alzheimer
disease incidence rates do not vary by sex in Rochester. Minn Arch Neurol
2002;59:1589-93. Back to cited text no. 6 |
7. |
Lang AE, Lozano AM. Parkinson's disease. First of two parts. N Engl
J Med 1998;339:1044-53. Back to cited text no. 7"> [PUBMED] [FULLTEXT] |
8. |
Lang AE, Lozano AM. Parkinson's disease. Second of two parts. N Engl
J Med 1998;339:1130-43. Back to cited text no. 8 |
9. |
Selkoe DJ. Cell biology of the amyloid beta-protein precursor and the
mechanism of Alzheimer's disease. Annu Rev Cell Biol 1994;10:373-403. Back to cited text no. 9 [PUBMED] [FULLTEXT] |
10. |
Kudo T, Iqbal K, Ravid R, Swaab DF, Grundke-Iqbal I. Alzheimer disease:
correlation of cerebro-spinal fluid and brain ubiquitin levels. Brain Res
1994;639:1-7. Back to cited text no. 10 [PUBMED] |
11. |
Suh YH, Checler F. Amyloid precursor protein, presenilins, and alpha-synuclein:
molecular pathogenesis and pharmacological applications in Alzheimer's
disease. Pharmacol Rev 2002;54:469-525. Back to cited text no. 11"> [PUBMED] [FULLTEXT] |
12. |
Ames BN, Shigenaga MK, Hagen TM. Oxidants, antioxidants, and the degenerative
diseases of aging. Proc Natl Acad Sci USA 1993;90:7915-22. Back to cited text no. 12"> [PUBMED] [FULLTEXT] |
13. |
Lafon-Cazal M, Pietri S, Culcasi M, Bockaert J. NMDA-dependent superoxide
production and neurotoxicity. Nature 1993;364:535-7. Back to cited text no. 13"> [PUBMED] [FULLTEXT] |
14. |
Kiyosawa H, Suko M, Okudaira H, Murata K, Miyamoto T, Chung MH, et al.
Cigarette smoking induces formation of 8-hydroxydeoxyguanosine, one of
the oxidative DNA damages in human peripheral leukocytes. Free Radic Res
Commun 1990;11:23-7. Back to cited text no. 14"> [PUBMED] |
15. |
Reznick AZ, Cross CE, Hu ML, Suzuki YJ, Khwaja S, Safadi A, et al. Modification
of plasma proteins by cigarette smoke as measured by protein carbonyl formation.
Biochem J 1992;286:607-11. Back to cited text no. 15"> [PUBMED] |
16. |
Dexter DT, Carter CJ, Wells FR, Javoy-Agid F, Agid Y, Lees A, et al.
Basal lipid peroxidation in substantia nigra is increased in Parkinson's
disease. J Neurochem 1989;52:381-9. Back to cited text no. 16"> [PUBMED] |
17. |
Dexter DT, Wells FR, Agid F, Agid Y, Lees AJ, Jenner P, et al. Increased
nigral iron content in postmortem parkinsonian brain. Lancet 1987;2:1219-20. Back to cited text no. 17"> [PUBMED] |
18. |
Gorell JM, Ordidge RJ, Brown GG, Deniau JC, Buderer NM, Helpern JA.
Increased iron-related MRI contrast in the substantia nigra in Parkinson's
disease. Neurology 1995;45:1138-43. Back to cited text no. 18"> [PUBMED] |
19. |
Ambani LM, Van Woert MH, Murphy S. Brain peroxidase and catalase in
Parkinson disease. Arch Neurol 1975;32:114-8. Back to cited text no. 19 [PUBMED] |
20. |
Kish SJ, Morito C, Hornykiewicz O. Glutathione peroxidase activity in
Parkinson's disease brain. Neurosci Lett 1985;58:343-6. Back to cited text no. 20 [PUBMED] |
21. |
Sofic E, Lange KW, Jellinger K, Riederer P. Reduced and oxidized glutathione
in the substantia nigra of patients with Parkinson's disease. Neurosci
Lett 1992;142:128-30. Back to cited text no. 21"> [PUBMED] |
22. |
Dexter DT, Holley AE, Flitter WD, Slater TF, Wells FR, Daniel SE, et
al. Increased levels of lipid hydroperoxides in the parkinsonian substantia
nigra: an HPLC and ESR study. Mov Disord 1994;9:92-7. Back to cited text no. 22 [PUBMED] |
23. |
Sanchez-Ramos J, Overvik E, Ames B. A marker of oxyradical-mediated
DNA damage (8-hydroxy-2'-deoxyguanosine) is increased in nigro-striatum
of Parkinson's disease brain. Neurodegeneration 1994;3:197-204. Back to cited text no. 23 |
24. |
Ebadi M, Srinivasan SK, Baxi MD. Oxidative stress and antioxidant therapy
in Parkinson's disease. Prog Neurobiol 1996;48:1-19. Back to cited text no. 24"> [PUBMED] [FULLTEXT] |
25. |
Polymeropoulos MH, Lavedan C, Leroy E, Ide SE, Dehejia A, Dutra A, et
al. Mutation in the alpha-synuclein gene identified in families with Parkinson's
disease. Science 1997;276:2045-7. Back to cited text no. 25"> [PUBMED] [FULLTEXT] |
26. |
Zhou W, Hurlbert MS, Schaack J, Prasad KN, Freed CR. Overexpression
of human alpha-synuclein causes dopamine neuron death in rat primary culture
and immortalized mesencephalon-derived cells. Brain Res 2000;866:33-43. Back to cited text no. 26"> [PUBMED] [FULLTEXT] |
27. |
Tabner BJ, Turnbull S, El-Agnaf OM, Allsop D. Formation of hydrogen
peroxide and hydroxyl radicals from A(beta) and alpha-synuclein as a possible
mechanism of cell death in Alzheimer's disease and Parkinson's disease.
Free Radic Biol Med 2002;32:1076-83. Back to cited text no. 27 |
28. |
Zhou W, Schaack J, Zawada WM, Freed CR. Oveexpression of human a-synuclein
causes dopamine neuron death in primary human mesencephalic culture. Brain
Res 2002;926:42-50. Back to cited text no. 28 |
29. |
Zhou W, Hurlbert MS, Schaack J, Prasad KN, Freed CR. Overexpression
of human alpha-synuclein causes dopamine neuron death in rat primary culture
and immortalized mesecephalon-derived cells. Brain Res 2000;866:33-43. Back to cited text no. 29 |
30. |
Giasson BI, Duda JE, Murray IV, Chen Q, Souza JM, Hurtig HI, et al.
Oxidative damage linked to neurodegeneration by selective alpha-synuclein
nitration in synucleinopathy lesions. Science 2000;290:985-9. Back to cited text no. 30 |
31. |
Kitada T, Asakawa S, Hattori N, Matsumine H, Yamamura Y, Minoshima S
et al. Mutations in the parkin gene cause autosomal recessive juvenile
parkinsonism. Nature 1998;392:605-8 Back to cited text no. 31 |
32. |
Harman D. A hypothesis on the pathogenesis of Alzheimer's disease. Ann
N Y Acad Sci 1996;786:152-68 Back to cited text no. 32 |
33. |
Shoffner JM, Brown MD, Torroni A, Lott MT, Cabell MF, Mirra SS et al.
Mitochondrial DNA variants observed in Alzheimer disease and Parkinson
disease patients. Genomics 1993;17:171-84 Back to cited text no. 33 |
34. |
Saraiva AA, Borges MM, Madeira MD, Tavares MA, Paula-Barbosa MM. Mitochondrial
abnormalities in cortical dendrites from patients with Alzheimer's disease.
J Submicrosc Cytol 1985;17:459-64 Back to cited text no. 34 |
35. |
Bowling AC, Beal MF. Bioenergetic and oxidative stress in neurodegenerative
diseases. Life Sci 1995;56:1151-71 Back to cited text no. 35 |
36. |
Mattson MP. Calcium and neuronal injury in Alzheimer's disease. Contributions
of beta-amyloid precursor protein mismetabolism, free radicals, and metabolic
compromise. Ann N Y Acad Sci 1994;747:50-76. Back to cited text no. 36 |
37. |
Gabuzda D, Busciglio J, Chen LB, Matsudaira P, Yankner BA. Inhibition
of energy metabolism alters the processing of amyloid precursor protein
and induces a potentially amyloidogenic derivative. J Biol Chem 1994;269:13623-8. Back to cited text no. 37 |
38. |
Brown AM, Kristal BS, Effron MS, Shestopalov AI, Ullucci PA, Sheu KF,
et al. Zn2+ inhibits alpha-ketoglutarate-stimulated mitochondrial respiration
and the isolated alpha-ketoglutarate dehydrogenase complex. J Biol Chem
2000;275:13441-7. Back to cited text no. 38 |
39. |
Gibson GE, Haroutunian V, Zhang H, Park LC, Shi Q, Lesser M, et al.
Mitochondrial damage in Alzheimer's disease varies with apolipoprotein
E genotype. Ann Neurol 2000;48:297-303. Back to cited text no. 39 |
40. |
Misonou H, Morishima-Kawashima M, Ihara Y. Oxidative stress induces
intracellular accumulation of amyloid beta-protein (Abeta) in human neuroblastoma
cells. Biochemistry 2000;39:6951-9. Back to cited text no. 40 |
41. |
Koppaka V, Axelsen PH. Accelerated accumulation of amyloid beta proteins
on oxidatively damaged lipid membranes. Biochemistry 2000;39:10011-6. Back to cited text no. 41 |
42. |
Schubert D, Behl C, Lesley R, Brack A, Dargusch R, Sagara Y, et al.
Amyloid peptides are toxic via a common oxidative mechanism. Proc Natl
Acad Sci USA 1995;92:1989-93. Back to cited text no. 42 |
43. |
Varadarajan S, Yatin S, Kanski J, Jahanshahi F, Butterfield DA. Methionine
residue 35 is important in amyloid beta-peptide-associated free radical
oxidative stress. Brain Res Bull 1999;50:133-41. Back to cited text no. 43 |
44. |
Behl C, Davis J, Cole GM, Schubert D. Vitamin E protects nerve cells
from amyloid beta protein toxicity. Biochem Biophys Res Commun 1992;186:944-50. Back to cited text no. 44 |
45. |
Pappolla MA, Chyan YJ, Omar RA, Hsiao K, Perry G, Smith MA, et al. Evidence
of oxidative stress and in vivo neurotoxicity of beta-amyloid in a transgenic
mouse model of Alzheimer's disease: a chronic oxidative paradigm for testing
antioxidant therapies in vivo. Am J Pathol 1998;152:871-7. Back to cited text no. 45 |
46. |
Zaman Z, Roche S, Fielden P, Frost PG, Niriella DC, Cayley AC. Plasma
concentrations of vitamins A and E and carotenoids in Alzheimer's disease.
Age Ageing 1992;21:91-4. Back to cited text no. 46 |
47. |
Smith MA, Kutty RK, Richey PL, Yan SD, Stern D, Chader GJ, et al. Heme
oxygenase-1 is associated with the neurofibrillary pathology of Alzheimer's
disease. Am J Pathol 1994;145:42-7. Back to cited text no. 47 |
48. |
Sims NR, Bowen DM, Neary D, Davison AN. Metabolic processes in Alzheimer's
disease: adenine nucleotide content and production of 14CO2 from [U-14C]glucose
in vitro in human neocortex. J Neurochem 1983;41:1329-34. Back to cited text no. 48 |
49. |
Martins RN, Harper CG, Stokes GB, Masters CL. Increased cerebral glucose-6-phosphate
dehydrogenase activity in Alzheimer's disease may reflect oxidative stress.
J Neurochem 1986;46:1042-5. Back to cited text no. 49 |
50. |
Saito K, Elce JS, Hamos JE, Nixon RA. Widespread activation of calcium-activated
neutral proteinase (calpain) in the brain in Alzheimer disease: a potential
molecular basis for neuronal degeneration. Proc Natl Acad Sci USA 1993;90:2628-32. Back to cited text no. 50 |
51. |
Zhou Y, Richardson JS, Mombourquette MJ, Weil JA. Free radical formation
in autopsy samples of Alzheimer and control cortex. Neurosci Lett 1995;195:89-92. Back to cited text no. 51 |
52. |
Koppal T. Peroxynitrite-mediated damage to brain membrane alterations
in Alzheimer's disease (AD). Soc Neurosci 1998;24:1217a. Back to cited text no. 52 |
53. |
Simic G, Lucassen PJ, Krsnik Z, Kruslin B, Kostovic I, Winblad B, et
al. nNOS expression in reactive astrocytes correlates with increased cell
death related DNA damage in the hippocampus and entorhinal cortex in Alzheimer's
disease. Exp Neurol 2000;165:12-26. Back to cited text no. 53 |
54. |
Conrad CC, Marshall PL, Talent JM, Malakowsky CA, Choi J, Gracy RW.
Oxidized proteins in Alzheimer's plasma. Biochem Biophys Res Commun 2000;275:678-81. Back to cited text no. 54 |
55. |
McIntosh LJ, Trush MA, Troncoso JC. Increased susceptibility of Alzheimer's
disease temporal cortex to oxygen free radical-mediated processes. Free
Radic Biol Med 1997;23:183-90. Back to cited text no. 55 |
56. |
Smith MA, Richey Harris PL, Sayre LM, Beckman JS, Perry G. Widespread
peroxynitrite-mediated damage in Alzheimer's disease. J Neurosci 1997;17:2653-7. Back to cited text no. 56 |
57. |
Sherrington R, Rogaev EI, Liang Y, Rogaeva EA, Levesque G, Ikeda M,
et al. Cloning of a gene bearing missense mutations in early-onset familial
Alzheimer's disease. Nature 1995;375:754-60. Back to cited text no. 57 |
58. |
Busciglio J, Hartmann H, Lorenzo A, Wong C, Baumann K, Sommer B, et
al. Neuronal localization of presenilin-1 and association with amyloid
plaques and neurofibrillary tangles in Alzheimer's disease. J Neurosci
1997;17:5101-7. Back to cited text no. 58 |
59. |
Dewji NN, Singer SJ. Genetic clues to Alzheimer's disease. Science 1996;271:159-60. Back to cited text no. 59 |
60. |
Hanson AJ, Prasad JE, Nahreini P, Andreatta C, Kumar B, Yan XD, et al.
The overexpression of amyloid precursor protein is associated with degeneration,
decreased viability, and increased damage caused by neurotoxins (Prostaglandins
A1 and E2, Hydrogen Peroxide and nitric oxide) in differentiated neuroblastoma
cells. J Neurosci Res 2003 (in press). Back to cited text no. 60 |
61. |
Le Couteur DG, Leighton PW, McCann SJ, Pond S. Association of a polymorphism
in the dopamine-transporter gene with Parkinson's disease. Mov Disord 1997;12:760-3. Back to cited text no. 61 |
62. |
Yamamoto M, Kondo I, Ogawa N, Asanuma M, Yamashita Y, Mizuno Y. Genetic
association between susceptibility to Parkinson's disease and alpha1-antichymotrypsin
polymorphism. Brain Res 1997;759:153-5. Back to cited text no. 62 |
63. |
Costa P, Checkoway H, Levy D, Smith-Weller T, Franklin GM, Swanson PD,
et al. Association of a polymorphism in intron 13 of the monoamine oxidase
B gene with Parkinson disease. Am J Med Genet 1997;74:154-6. Back to cited text no. 63 |
64. |
Takakubo F, Yamamoto M, Ogawa N, Yamashita Y, Mizuno Y, Kondo I. Genetic
association between cytochrome P450IA1 gene and susceptibility to Parkinson's
disease. J Neural Transm Gen Sect 1996;103:843-9. Back to cited text no. 64 |
65. |
Marx J. New gene tied to common form of Alzheimer's. Science 1998;281:507-9. Back to cited text no. 65 |
66. |
McConnell LM, Koenig BA, Greely HT, Raffin TA. Genetic testing and Alzheimer
disease: has the time come? Alzheimer Disease Working Group of the Stanford
Program in Genomics, Ethics & Society. Nat Med 1998;4:757-9. Back to cited text no. 66 |
67. |
Lam YA, Pickart CM, Alban A, Landon M, Jamieson C, Ramage R, et al.
Inhibition of the ubiquitin-proteasome system in Alzheimer's disease. Proc
Natl Acad Sci USA 2000;97:9902-6. Back to cited text no. 67 |
68. |
McMillan PJ, Leverenz JB, Dorsa DM. Specific downregulation of presenilin
2 gene expression is prominent during early stages of sporadic late-onset
Alzheimer's disease. Brain Res Mol Brain Res 2000;78:138-45. Back to cited text no. 68 |
69. |
Fraser P, Tu G, Levesque G. Molecular genetics of the presenilins in
Alzheimer's disease. In: Christen Y, editor. Presenilins and Alzheimer's
Disease. Berlin: Springer-Verlag; 1998. pp. 1-10. Back to cited text no. 69 |
70. |
Checler F, da Costa CA, Ancolio K, Chevallier N, Lopez-Perez E, Marambaud
P. Role of the proteasome in Alzheimer's disease. Biochim Biophys Acta
2000;1502:133-8. Back to cited text no. 70 |
71. |
Rockwell P, Yuan H, Magnusson R, Figueiredo-Pereira ME. Proteasome inhibition
in neuronal cells induces a proinflammatory response manifested by upregulation
of cyclooxygenase-2, its accumulation as ubiquitin conjugates, and production
of the prostaglandin PGE(2). Arch Biochem Biophys 2000;374:325-33. Back to cited text no. 71 |
72. |
Gregori L, Hainfeld JF, Simon MN, Goldgaber D. Binding of amyloid beta
protein to the 20 S proteasome. J Biol Chem 1997;272:58-62. Back to cited text no. 72 |
73. |
Lopez Salon M, Morelli L, Castano EM, Soto EF, Pasquini JM. Defective
ubiquitination of cerebral proteins in Alzheimer's disease. J Neurosci
Res 2000;62:302-10. Back to cited text no. 73 |
74. |
Nahreini P, Andreatta C, Prasad KN. Proteasome activity is critical
for the cAMP-induced differentiation of neuroblastoma cells. Cell Mol Neurobiol
2001;21:509-21. Back to cited text no. 74 |
75. |
Wang GP, Khatoon S, Iqbal K, Grundke-Iqbal I. Brain ubiquitin is markedly
elevated in Alzheimer disease. Brain Res 1991;566: Back to cited text no. 75 |
76. |
146-51. Back to cited text no. 76"> [PUBMED] |
77. |
Goedert M, Jakes R, Crowther RA, Six J, Lubke U, Vandermeeren M, et
al. The abnormal phosphorylation of tau protein at Ser-202 in Alzheimer
disease recapitulates phosphorylation during development. Proc Natl Acad
Sci U S A 1993;90:5066-70. Back to cited text no. 77 |
78. |
Borroni B, Colciaghi F, Lenzi GL, Caimi L, Cattabeni F, Di Luca M, et
al. High cholesterol affects platelet APP processing in controls and in
AD patients. Neurobiol Aging 2003;24:631-6. Back to cited text no. 78 |
79. |
Pappolla MA, Bryant-Thomas TK, Herbert D, Pacheco J, Fabra Garcia M
, Manjon M, et al. Mild hypercholesterolemia is an early risk factor for
the development of Alzheimer amyloid pathology. Neurology 2003;61:199-205. Back to cited text no. 79 |
80. |
Austen BM, Sidera C, Liu, C. Frears E. The role of ontracellular cholesterol
on the processing of the amyloid precursor protein. J Nutr Health Aging
2003;7:31-6. Back to cited text no. 80 |
81. |
Sparks DL, Martin TA, Gross DR, Hunsaker JC 3rd. Link between heart
disease, cholesterol, and Alzheimer's disease: a review. Microsc Res Tech
2000;50:287-90. Back to cited text no. 81 |
82. |
Jick H, Zornberg GL, Jick SS, Seshadri S, Drachman DA. Statins and the
risk of dementia. Lancet 2000;356:1627-31. Back to cited text no. 82 |
83. |
Refolo LM, Malester B, LaFrancois J, Bryant-Thomas T, Wang R, Tint GS,
et al. Hypercholesterolemia accelerates the Alzheimer's amyloid pathology
in a transgenic mouse model. Neurobiol Dis 2000;7:321-31. Back to cited text no. 83 |
84. |
Simons M, Keller P, De Strooper B, Beyreuther K, Dotti CG, Simons K.
Cholesterol depletion inhibits the generation of beta-amyloid in hippocampal
neurons. Proc Natl Acad Sci USA 1998;95:6460-4. Back to cited text no. 84 |
85. |
Kumar B, Andreatta C, Koustas WT, Cole WC, Edwards-Prasad J, Prasad
KN. Mevastatin induces degeneration and decreases viability of cAMP-induced
differentiated neuroblastoma cells in culture by inhibiting proteasome
activity, and mevalonic acid lactone prevents these effects. J Neurosci
Res 2002;68:627-35. Back to cited text no. 85 |
86. |
Sparks DL, Lochhead J, Horstman D, Wagoner T, Martin T. Water quaility
has a pronounced effect on cholesterol-induced accumulation of Alzheimer
amyloid beta (Abeta) in rabbit brain. J Alzheimers Dis 2002;4:523-9. Back to cited text no. 86 |
87. |
Vatassery GT, Brin MF, Fahn S, Kayden HJ, Traber MG. Effect of high
doses of dietary vitamin E on the concentrations of vitamin E in several
brain regions, plasma, liver, and adipose tissue of rats. J Neurochem 1988;51:621-3. Back to cited text no. 87 |
88. |
Pillai SR, Traber MG, Steiss JE, Kayden HJ, Cox NR. Alpha-tocopherol
concentrations of the nervous system and selected tissues of adult dogs
fed three levels of vitamin E. Lipids 1993;28:1101-5. Back to cited text no. 88 |
89. |
Cadet JL, Katz M, Jackson-Lewis V, Fahn S. Vitamin E attenuates the
toxic effects of intrastriatal injection of 6-hydroxydopamine (6-OHDA)
in rats: behavioral and biochemical evidence. Brain Res 1989;476:10-5. Back to cited text no. 89 |
90. |
Perumal AS, Gopal VB, Tordzro WK, Cooper TB, Cadet JL. Vitamin E attenuates
the toxic effects of 6-hydroxydopamine on free radical scavenging systems
in rat brain. Brain Res Bull 1992;29:699-701. Back to cited text no. 90 |
91. |
Schubert D, Kimura H, Maher P. Growth factors and vitamin E modify neuronal
glutamate toxicity. Proc Natl Acad Sci USA 1992;89:8264-7. Back to cited text no. 91 |
92. |
O'hare E, McDiad D, Wilson R, Kennedy F, Kim E. Chronic alpha-tocopherol
administrtion protects against behavioral impairment induced following
intra-hipocampal Aß injection. Presented at Society for Neuroscience Annual
Meeting: 2000. pp. 1788a. Back to cited text no. 92 |
93. |
Martin A, Cuervo A, Dice J, Joseph J. Effect of vitamin C on intracellular
protein turnover in human glial cells. Presented at Society for Neuroscience
Annual Meeting: 2000. pp. 1830a. Back to cited text no. 93 |
94. |
Fahns S. An open trial of high-dosage antioxidants in early Parkinson's
disease. Am J Clin Nutr 1991;53:380S-2S. Back to cited text no. 94 |
95. |
Effects of tocopherol and deprenyl on the progression of disability
in early Parkinson's disease. The Parkinson Study Group. N Engl J Med 1993;328:176-83. Back to cited text no. 95 |
96. |
Sano M, Ernesto C, Thomas RG, Klauber MR, Schafer K, Grundman M, et
al. A controlled trial of selegiline, alpha-tocopherol, or both as treatment
for Alzheimer's disease. The Alzheimer's Disease Cooperative Study. N Engl
J Med 1997;336:1216-22. Back to cited text no. 96 |
97. |
Ingold KU, Burton GW, Foster DO, Hughes L, Lindsay DA, Webb A. Biokinetics
of and discrimination between dietary RRR- and SRR-alpha-tocopherols in
the male rat. Lipids 1987;22:163-72. Back to cited text no. 97 |
98. |
Prasad KN, Kumar B, Yan XD, Hanson AJ, Cole WC. Alpha-Tocopheryl succinate,
the most effective form of Vitamin E for adjuvant cancer treatment: A Review.
J Am Coll Nutr 2003;22:108-17. Back to cited text no. 98 |
99. |
Krinsky NI. Antioxidant functions of carotenoids. Free Radic Biol Med
1989;7:617-35. Back to cited text no. 99 |
100. |
Zhang LX, Cooney RV, Bertram JS. Carotenoids up-regulate connexin43
gene expression independent of their provitamin A or antioxidant properties.
Cancer Res 1992;52:5707-12. Back to cited text no. 100 |
101. |
Vile GF, Winterbourn CC. Inhibition of adriamycin-promoted microsomal
lipid peroxidation by beta-carotene, alpha-tocopherol and retinol at high
and low oxygen partial pressures. FEBS Lett 1988;238:353-6. Back to cited text no. 101 |
102. |
McCay PB. Vitamin E: interactions with free radicals and ascorbate.
Annu Rev Nutr 1985;5:323-40. Back to cited text no. 102 |
103. |
Perry TL, Godin DV, Hansen S. Parkinson's disease: a disorder due to
nigral glutathione deficiency? Neurosci Lett 1982;33:305-10. Back to cited text no. 103 |
104. |
Witschi A, Reddy S, Stofer B, Lauterburg BH. The systemic availability
of oral glutathione. Eur J Clin Pharmacol 1992;43:667-9. Back to cited text no. 104 |
105. |
Niki E. Mechanisms and dynamics of antioxidant action of ubiquinol.
Mol Aspects Med 1997;18:S63-70. Back to cited text no. 105 |
106. |
Stoyanovsky DA, Osipov AN, Quinn PJ, Kagan VE. Ubiquinone-dependent
recycling of vitamin E radicals by superoxide. Arch Biochem Biophys 1995;323:343-51. Back to cited text no. 106 |
107. |
Chen RS, Huang CC, Chu NS. Coenzyme Q10 treatment in mitochondrial encephalomyopathies.
Short-term double-blind, crossover study. Eur Neurol 1997;37:212-8. Back to cited text no. 107 |
108. |
Birkmayer W, Birkmayer JGD, Vrecko C, Paletta B, Reschenhofer E, Ott
E. Nicotinamide adenine dinucleotide (NADH) as medication for Parkinson's
disease. Experience with 415 patients. New Trends Clin Neuropharmacol 1990;4:7-24. Back to cited text no. 108 |
109. |
Birkmayer W, Birkmayer JG, Vrecko K, Paletta B. The clinical benefit
of NADH as stimulator of endogenous L-dopa biosynthesis in parkinsonian
patients. In: Youdim MBH, editor. Advances in Neurology, Parkinson's Disease:
Anatomy, Pathology, and Therapy. New York: Raven Press; 1990. pp. 545-9. Back to cited text no. 109 |
110. |
Vrecko K, Birkmayer JG, Krainz J. Stimulation of dopamine biosynthesis
in cultured PC 12 phaeochromocytoma cells by the coenzyme nicotinamide
adeninedinucleotide (NADH). J Neural Transm Park Dis Dement Sect 1993;5:147-56. Back to cited text no. 110 |
111. |
Birkmayer JG. Coenzyme nicotinamide adenine dinucleotide: new therapeutic
approach for improving dementia of the Alzheimer type. Ann Clin Lab Sci
1996;26:1-9. Back to cited text no. 111 |
112. |
Regland B, Gottfries CG, Oreland L. Vitamin B12-induced reduction of
platelet monoamine oxidase activity in patients with dementia and pernicious
anaemia. Eur Arch Psychiatry Clin Neurosci 1991;240:288-91. Back to cited text no. 112 |
113. |
Nadeau A, Roberge AG. Effects of vitamin B12 supplementation on choline
acetyltransferase activity in cat brain. Int J Vitam Nutr Res 1988;58:402-6. Back to cited text no. 113 |
114. |
Ikeda T, Yamamoto K, Takahashi K, Kaku Y, Uchiyama M, Sugiyama K, et
al. Treatment of Alzheimer-type dementia with intravenous mecobalamin.
Clin Ther 1992;14:426-37. Back to cited text no. 114 |
Copyright 2003 - Journal of Postgraduate Medicine. Online full-text also available
at http://www.jpgmonline.com/
The following images related to this document are available:
Photo images
[jp03065t1.jpg]
[jp03065t2.jpg]
|