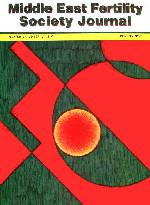
|
Middle East Fertility Society Journal
Middle East Fertility Society
ISSN: 1110-5690
Vol. 9, Num. 3, 2004, pp. 187-197
|
Middle East Fertility Society Journal, Vol. 9, No. 3, 2004, pp.
187-197
REVIEW
Oxidants and antioxidants in human fertility
Ashok Agarwal, Ph.D., HCLD., Shyam S.R. Allamaneni, M.D.
Center for Advanced Research in Human
Reproduction, Infertility and Sexual Function, Glickman Urological Institute
and Department of Obstetrics and Gynecology, Cleveland Clinic Foundation,
Cleveland, Ohio.
Correspondence and reprint requests: Dr. Ashok Agarwal, Director, Center for Advanced Research in Human, Reproduction, Infertility, and Sexual Function, Glickman Urological Institute and Department of Obstetrics-Gynecology, The Cleveland Clinic Foundation, 9500 Euclid Avenue, Desk A19.1, Cleveland, OH 44195, USA (FAX: 216-445-6049; e-mail: agarwaa@ccf.org).
Received on June 15, 2004;
revised and accepted on August 29, 2004
Code Number: mf04034
ABSTRACT
Oxidants are highly unstable molecules
that attack every chemical substance they come into contact. Oxidants modify
the macromolecules both structurally and functionally. Body has defense
mechanisms against oxidants in the form of both enzymatic and non-enzymatic
antioxidants. Reactive oxygen species (ROS) are a group of oxidants formed
during oxygen metabolism. ROS appears to be involved in the pathogenesis of
many human diseases. In reproductive medicine, ROS have both physiological and
pathological role in male and female reproduction. Oxidative stress develops
when the generation of ROS overwhelms the scavenging capacity of antioxidants.
Oxidative stress causes damage to spermatozoa, oocyte and embryos. It appears
to play a role in both natural and in vitro fertilization and pregnancy. The
patients with oxidative stress may benefit from the strategies to reduce
oxidative stress and treatment with antioxidants.
Key words: Oxidants, antioxidants, infertility - male & female, spermatozoa, reactive
oxygen species, free radicals.
INTRODUCTION
Infertility can be defined as a lack of pregnancy after one year of regular unprotected intercourse. Approximately 15%-20% of couples of reproductive age are infertile, which can be attributed equally to both male and female factors. Recent research on the role of reactive oxygen species (ROS) in human infertility has received a great deal of interest from the scientists and medical practitioners (1-3). This review summarizes the latest consensus on the role of ROS in human reproduction. It should help clarify the role of ROS in human fertility and may lead into the development of newer therapeutic approaches to treat infertility.
Oxidants
Reactive oxygen species (ROS) are oxygen-derived molecules, which are formed as intermediary products and are a class of powerful oxidants in the human body. ROS include superoxide anion (O2((), hydrogen peroxide (H2O2) and hydroxyl radical (OH.). Some cells posses specific mechanisms to produce ROS that are required for cellular functions in low concentrations (4). Aerobic environment is a constant source of ROS through in vivo mechanisms such as electron leakage during biologic oxidations, and by physical activation of oxygen by external agents such as irradiation, e.g. UV sunlight. ROS are characterized by their ability to react with any molecule they come in contact and modify it oxidatively. The modification may result in structural and functional alterations and impair many cellular processes. Depending on their tissue concentration they can either exert beneficial physiologic effects (e.g. play role in fertilization process) or pathological damage to cellular components, including lipids, proteins and nucleic acids (5).
Antioxidants
Organisms have developed efficient protective mechanisms against excessive accumulation of ROS. ROS are neutralized by an elaborate antioxidant defense system consisting of enzymes such as catalase, superoxide dismutase and glutathione peroxidase/reductase, and numerous non-enzymatic antioxidants such as vitamin C, vitamin E, vitamin A, pyruvate, glutathione, taurine and hypotaurine (6). In a healthy body, pro-oxidants and antioxidants maintain a ratio and a shift in this ratio towards pro-oxidants gives rise to oxidative stress. This oxidative stress may be either mild or severe depending on the extent of shift. Whenever ROS levels become pathologically elevated, antioxidants begin to work and help minimize the oxidative damage, repair it or prevent it altogether. The male and female genital tracts are rich in both enzymatic and non-enzymatic antioxidants (7-10). Vitamins C and E act as chain-breaking antioxidants and thus prevent the propagation of peroxidative process.
OXIDANTS AND PATHOLOGICAL MECHANISMS OF CELL INJURY
Lipid peroxidation
ROS can attack polyunsaturated fatty acids in the cell membrane leading to a chain of chemical reactions called lipid peroxidation. Fatty acid breakdown results in the formation of various oxidatively modified products, which are toxic to cells and are finally converted into stable end products. The spermatozoal membrane contains large amounts of polyunsaturated fatty acids (11), which maintain its fluidity. Peroxidation of these fatty acids leads to the loss of membrane fluidity and a reduction in the activity of membrane enzymes and ion channels. As a result, the normal cellular mechanisms that are required for fertilization are inhibited. It is possible to measure the extent of peroxidative damage by estimating the stable end products of lipid peroxidation such as malondialdehyde (5).
DNA damage
Susceptibility of DNA to oxidative damage is indicated by the presence of oxidatively modified substances like 8-hydroxy-2-deoxyguanosine. Deoxyribonucleic acid bases and phosphodiester backbones are sites that are susceptible to peroxidative damage. High levels of ROS mediate the DNA fragmentation that is commonly observed in the spermatozoa of infertile men (12, 13). Normally, sperm DNA is protected from oxidative insult by its specific compact organization and by antioxidants in the seminal plasma. Spermatozoa are unique in that they cannot repair DNA and depend on the oocyte for repair after fertilization (14). Various types of DNA abnormalities occur in sperm that have been exposed to ROS artificially. These abnormalities include base modification, production of base-free sites, deletions, frame shifts, DNA cross-links and chromosomal rearrangements (15, 16). Patients with high levels of oxidative stress in their seminal fluid were found to have sperm with multiple single and double DNA strand breaks (17). A biomarker for oxidative DNA damage, 8-hydroxy-2-deoxyguanosine, can be used to determine the extent of ROS-induced DNA damage.
Apoptosis
ROS may also initiate a chain of reactions that ultimately lead to apoptosis.
Apoptosis is a natural process in which the body removes old and senescent
cells; it is a process of programmed cell death. In human germ cells, apoptosis
may help remove abnormal germ cells and prevent their overproduction. Multiple
extrinsic and intrinsic cell factors control the process of apoptosis (3).
In a study from our center, levels of ROS were positively associated with apoptosis
in mature spermatozoa. Levels of caspases, which are proteases involved in
apoptosis, correlated with levels of ROS. Our results also showed that apoptosis
could be induced in cell cultures with H2O2, which further supports the theory
that ROS is involved in apoptosis. The process of apoptosis may also be accelerated
by ROS-induced DNA damage and ultimately may lead to a decline in sperm count
(6).
OXIDATIVE STRESS MEASUREMENT
Oxidative stress can be estimated directly or indirectly. The direct measurement of ROS is by using electron spin resonance method and is used sparingly in reproductive medicine. Indirect tests measure oxidatively modified products. Chemiluminescence is a common method used and is based on emission of light on chemiluminescent reaction between ROS and reagent (luminal/lucigenin). The amount of light emitted is quantified and measured by a luminometer.
Lipid peroxidation end products like malondialdehyde, lipid hydroperoxides, and conjugated dienes are commonly used to assess the oxidative stress. Other methods are measurement of protein and DNA oxidation products, and changes in status of antioxidants. Flow cytometry is also being used to measure the individual ROS radicals (18).
ROS-TAC score
It is a concept proposed by our group to represent the oxidative stress status of individual more accurately. This score accommodate for the variations in both ROS and TAC (total antioxidant capacity) values (19). Fertile men tend to have high ROS-TAC scores whereas infertile men generally have significantly lower scores. ROS levels can also be measured directly in neat semen, thereby offering yet another measure of oxidative stress.
Oxidative Stress and Male Infertility
The presence of free radicals in the spermatozoa was reported by MacLeod 50 years ago (20). Because spermatozoa lack cytoplasmic enzymes, they often are unable to prevent oxidative damage by these free radicals. This is one of the features that make spermatozoa highly susceptible to peroxidative damage. Most cytoplasmic enzymes are extruded during the final stages of the sperm maturation process, which enables sperm to attain their characteristic morphology (21). Nature compensated for this deficiency by providing an array of antioxidants in the seminal plasma.
Sources of oxidants
Morphologically abnormal spermatozoa and leukocytes are the major sources of ROS in the male reproductive tract. Even though mature spermatozoa may not produce pathologically significant levels of ROS, oxidative damage may occur in the epididymis and seminiferous tubules where they are in close contact with the immature, ROS producing spermatozoa and leukocytes (22).
ROS production is elevated in patients who have a large percentage of spermatozoa with excess residual cytoplasm in the midpiece (22). Excess residual cytoplasm contains enzymes such as glucose-6-phosphate dehydrogenase and creatine phosphokinase, which are linked with generation of ROS and defective sperm function. ROS may be generated at the level of plasma membrane (NADPH-oxidase system) (23) or mitochondria (NADH-dependent oxido-reductase) (24). Human spermatozoa generate O2(( (25), which spontaneously or enzymatically dismutates to H2O2. In the presence of metal ions (iron)- O2(( and H2O2 together produces the more harmful oxidant, OH..
Neutrophils and macrophages are the major source of oxidants in the reproductive tract (26, 27). During inflammation and infection, activated leukocytes can produce significantly higher amounts of ROS than non-activated leukocytes (28). The ROS production in leukocytes is through NADPH oxidase enzyme. Even though ROS is released as part of defense mechanism in to the reproductive tract, it can damage surrounding spermatozoa, especially when antioxidant systems are overwhelmed. The importance of leukocyte contamination in producing ROS is well observed in Percoll-washed spermatozoa where a small number of leukocytes produce ROS. Increased levels of seminal leukocytes may also stimulate human spermatozoa to produce ROS. Such stimulation may be mediated via direct cell-cell contact or by soluble products released by leukocytes (27).
Mechanism of loss of sperm function
The mechanism of loss of sperm function by ROS appears to be multifold (29). ROS may affect the quality and number of spermatozoa reaching the ovum in the female reproductive tract. In addition, ROS impair the fertilization process by preventing the initiation of sperm-oocyte fusion events (14). Finally, ROS can impair embryo development and affect the health of offspring by damaging sperm DNA (16).
Impairment of standard semen parameters
Motility is a very important attribute unique to spermatozoa in entire human cells. Motility is indispensable to the spermatozoa, as it has to travel the female reproductive tract to reach the site of fertilization. Studies found that levels of ROS correlate with motility of spermatozoa (30, 31). In vitro studies showed that the impaired motility may be a temporary event or permanent phenomena. Excessive ROS causes ATP to deplete rapidly resulting in decreased phosphorylation of axonemal proteins and cause transient impairment of motility (32). Peroxidative damage to the sperm membrane and axonemal proteins appears to be the cause of permanent impairment in sperm motility.
ROS appears to play a role in the apoptosis of spermatozoa by activating caspases. Under normal conditions, abnormal sperm undergo apoptosis, which minimizes their presence in the semen. The severity of oligozoospermia has been correlated with excessive levels of ROS (33). ROS may stimulate the process of apoptosis, resulting in the death of spermatozoa and decreased sperm count (6). Patients with a low sperm count have a reduced chance of initiating a pregnancy. In view of recent reports of declining sperm counts during the last several decades, a possible association between oxidative damage to spermatozoa and declining sperm count in the general population is postulated (34).
Impairment of sperm-oocyte fusion
A minimal amount of ROS is required for the normal sperm-oocyte fusion. Spermatozoa and oocyte has inbuilt mechanism to prevent excessive production of ROS at the time of sperm-oocyte fusion, this may be by the release of SOD (superoxide dismutase) (35). If there is an abnormality in the production of SOD, ROS generation can continue uninterruptedly and damage both spermatozoa and oocyte. The affect of ROS on sperm fertilizing capacity cannot be quantified by measuring routine semen parameters. It is possible that the levels of ROS needed to impair sperm-oocyte fusion events are lower than those required to affect sperm motility. The inability of sperm to fuse with an oocyte appears to be due to the effects of ROS on the sperm membrane. The lipid peroxidation process results in loss of membrane fluidity due to disorganization of membrane architecture and reduction in the activity of membrane enzymes and ion channels. As a result, spermatozoa are unable to initiate the necessary biochemical reactions associated with acrosome reaction, zona pellucida binding and oocyte penetration (36, 37).
Sperm DNA damage
Sperm DNA contributes the half of genomic material to the offspring. Thus, normal sperm genetic material is required for fertilization, embryo and fetus development and postnatal child well being (16, 38). A recent study showed decreasing likelihood of pregnancy with increasing levels of 8-hydroxy-2-deoxyguanosine, an indicator of oxidative damage to DNA (39). The percentage of sperm with DNA damage is negatively correlated with the fertilization rate (12). Oocytes can repair DNA damage to some extent, but when the damage is severe, embryo death and miscarriages can occur. The affect of ROS on DNA integrity has become the focus of recent attention due to widespread use of assisted reproduction techniques (ART) such as intracytoplasmic injection (ICSI). In natural pregnancy, oxidative damage to the sperm membrane may ensure that spermatozoa with damaged DNA lose their ability to fertilize an oocyte. However, sperm with DNA damage can potentially be injected into an oocyte in the ICSI resulting in fertilization and pregnancy which may progress to live birth with congenital abnormalities (34).
Male infertility, oxidative stress and clinical applications
For the clinician it is important to know the clinical conditions in which oxidative stress may play a role in the etiology of infertility. Many clinical conditions were found to be associated with increased oxidative stress (33). Infections and inflammations involving the male reproductive tract are obvious conditions associated with oxidative stress in view of excessive generation of ROS by leukocytes (40-42). Very high percentage of spinal cord injury patients were reported to have elevated levels of oxidative stress (43, 44). Mechanism of infertility in patients with varicocele is poorly understood and ROS is postulated as a possible mediator (45, 46). Elevated levels of ROS and depressed levels of TAC were associated with varicocele (47-49). Patients who underwent vasectomy reversal also had high levels of reactive oxygen species (50, 51). A history of smoking was associated with high levels of oxidative stress (52).
ROLE OF OXIDANTS IN FEMALE INFERTILITY
The role of oxidative stress in female reproductive diseases and infertility is under intense investigations. Many studies reported the presence of oxidative and antioxidant systems in various female reproductive tissues (53-57). ROS appears to have physiological role in female reproductive tract in many different processes such as: oocyte maturation, fertilization, luteal regression, and endometrial shedding (58, 59). ROS levels in follicular fluid may be used as markers for predicting the success of in vitro fertilization (IVF)(3).
Whenever there is imbalance in the levels of ROS and antioxidants- damage can occur to oocytes and embryos through various pathological mechanisms described previously. Oxidative stress can affect the female fertility potential in number of ways. It may affect the ovulation, fertilization, embryo development and implantation.
The sources of ROS in Graffian follicle may be macrophages, neutrophils and granulosa cells. Follicular fluid contains high levels of antioxidants, which protect oocytes from ROS-induced damage. Significantly lower selenium levels were detected in follicular fluid of patients with unexplained infertility compared with those with tubal infertility or couples with male factor infertility (60). Another study reported that baseline TAC levels were higher in follicles whose oocytes fertilized successfully (61).
Elevated levels of ROS in peritoneal fluid may be the cause of infertility in some women who do not have any other obvious cause. Elevated levels can damage the ovum after its release from the ovary, the zygote/embryo and most importantly, spermatozoa. As discussed previously, spermatozoa are very sensitive to oxidative stress. Studies have compared ROS levels in peritoneal fluid between women undergoing laparoscopy for infertility evaluation and fertile women undergoing tubal ligation. ROS levels in the peritoneal fluid were significantly higher in the patients with idiopathic infertility compared with the fertile women (57, 62). High levels of malondialdehyde and low levels of antioxidants in the peritoneal fluid were reported in patients with unexplained infertility compared to controls (63).
Do oxidants have a role in pathogenesis of the endometriosis?
Oxidative stress is postulated as one of the possible mechanism of endometriosis. (64). The endometrial tissue has multiple cells like macrophages, red blood cells, which can generate ROS. Studies of women with endometriosis have suggested that peritoneal macrophages are responsible for increased production of ROS or increased expression of xanthine oxidase in endometrial cells (65, 66). High levels of oxidatively modified substances in peritoneal fluid and ectopic endometrial tissue were reported (67). Altered expression of defensive antioxidant enzymes and low levels of vitamin E were reported in patients with endometriosis (68). However, ROS levels showed no difference between healthy women and those with endometriosis (57, 62). Thus, more studies are needed to clearly understand the role of ROS in endometriosis.
Oxidants and hydrosalpingeal fluid
Hydrosalpinx has been associated with decreased outcome after IVF. But, the toxic agent associated with hydrosalpinx is not clearly known (69). A study from our center demonstrated the presence of ROS, antioxidants and lipid peroxidation products in hydrosalpingeal fluid (HSF) (70). We found that mouse embryo blastocyst development rate was higher when they were incubated with HSF containing low ROS levels than when incubated with HSF without ROS. These low ROS levels may in fact represent the physiological levels and thus account for observed positive relationship between ROS levels and blastocyst development rate.
Effect of Oxidants on Embryo Growth
Oxidative stress appears to have a detrimental effect on the development of embryo. ROS may originate from embryo metabolism and from the surrounding environment (71, 72). ROS not only alters most types of cellular molecules but also induces early embryonic developmental block and retardation (56). High levels of ROS and apoptosis were reported in fragmented embryos compared to non-fragmented embryos (73). Multiple mechanisms of embryo protection against ROS exist (74, 75).
According to a study from our center, ROS levels in day 1 culture media can help predict the success of fertilization, embryo development and pregnancy (76). In our study, ROS levels in Day 1 culture media correlated well with fertilization and embryo development in patients undergoing IVF and ICSI. They also correlated with pregnancy in ICSI but not in IVF.
Effects of Oxidants on In Vitro Reproduction
DNA damage induced by oxidative stress has important clinical implications in the context of assisted reproduction. Spermatozoa selected for ART most likely originate from an environment experiencing OS, and a large percentage of these sperm may have damaged DNA (2). There is a strong possibility that spermatozoa with damaged DNA may be used during ART (16), which can negatively affect the ART success rate and increase the risk of spontaneous abortion or offspring with genetic disorders. ROS levels in mature spermatozoa correlate significantly with the fertilizing potential of spermatozoa (77, 78). Estimating ROS levels may help predict the success rate of assisted reproduction procedures.
ROS and Sperm Preparation
A possible source of ROS in ART media is during the preparation of semen. Sperm preparation is necessary to enhance and maintain sperm quality and function after ejaculation before the semen specimen can be used for ART procedures (79). The production of ROS may be due to either 1) activation of spermatozoa by centrifugation process, 2) absence of antioxidant rich seminal plasma, or 3) because of minimal contamination of ROS produced by leukocytes and abnormal spermatozoa. The small amount of ROS produced may not decrease motility but can still cause DNA damage (29). A proper sperm preparation method should be selected so as to decrease the production of ROS.
THERAPEUTIC INTERVENTION AGAINST OXIDANTS
General treatment strategies
In both male and female reproduction, oxidative stress appears to be due to increased generation of ROS rather than a depletion of antioxidants. It is important to identify the source of increased ROS generation (80). The underlying etiological factor for abnormal leukocyte infiltration (e.g. leukocytospermia, inflammation, infection, smoking) should be determined. Patients with history of smoking should be advised to stop smoking. Any exposure to drugs, toxic substances and radiation should be checked and patients advised to stop exposure to them. Infections of the reproductive tract should be treated with appropriate antibiotics.
Initially, specific therapeutic options directed against the etiological cause of raised ROS should be tried. Patients with reproductive tract infection should be treated with antibiotics. Anti-inflammatory agents may help patients with persistent leukocytospermia and elevated levels of cytokines. Antioxidant supplementation may or may not be effective depending on the pathology of the infertility. After treating the primary cause (such as varicocele), patients can be advised to take antioxidant supplementation. Antioxidants can be started directly when a specific etiology cannot be identified (idiopathic infertility).
Male infertility
Semen analysis should be repeated after a full spermatogenic cycle in those men showing large number of abnormal spermatozoa with excessive cytoplasm in the mid piece during a routine analysis. This can help distinguish between a temporary disturbance in spermatogenesis and a permanent defect in spermatogenesis. Varicocelectomy may remove an unknown stimulus of ROS generation. Even though there is no definitive consensus on the use of antioxidants, many in vitro and in vivo studies have shown that they improve semen quality and fertility (80).
Some studies showed improvement in terms of pregnancy rate after antioxidants supplementation. Oral vitamin E is an antioxidant favored by many researchers and clinicians. Many formulations of vitamin E are available as oral supplements. Oral administration of 300 mg twice a day of vitamin E in a randomized double blind placebo controlled trial showed significant improvement of pregnancy rates (21%; 11/52) in infertile (asthenozoospermic) patients, while resulting in lack of pregnancies in the placebo group. This study also found significant improvement in sperm motility, and reduced lipid peroxidation levels after vitamin E supplementation (81). A combination of vitamin E and selenium in oligoasthenoteratozoospermic (OAT) patients resulted in significant improvement in sperm motility, viability and morphology (82).
Treatment may be more appropriate if antioxidants are given to the patients with raised ROS levels. In one such study, when infertile patients with high ROS production were treated with GSH (reduced glutathione) (600 mg), a positive effect on sperm motility, on sperm morphology and significantly reduced lipid peroxidation levels were observed after 30 days of treatment (83).
The efficacy of antioxidants may be evaluated by measuring ROS levels during treatment. But, from a clinical point of view, improved pregnancy rate may be a more relevant end point. The combination therapy of vitamin E with N-acetyl-cysteine (NAC) or vitamins A plus E and essential fatty acids significantly reduced ROS and improved pregnancy rates (84). Several studies have demonstrated the ability of carnitines (L-carnitine 1 g and acetyl-carnitine 0.5 g twice a day) to significantly reduce the ROS production and increase the chances of a couple to become pregnant (85-87).
The importance of studying sperm function tests or pregnancy rate is emphasized by observation that the improvement in the fertilization rate is not always accompanied by a concomitant improvement in sperm parameters. Treatment of subfertile patients with low fertilization rates after ICSI with oral coenzyme Q10 60 mg/day for 103 days, improved fertilization rate in ICSI, although no significant improvement was observed in sperm parameters (88).
Some studies reported antioxidants effect in terms of their ability to decrease sperm DNA damage levels. Oral administration of 200 mg of vitamin C, 200 mg of vitamin E and 400 mg of GSH for 2 months significantly improved serum levels of antioxidants and relatively decreased sperm DNA damage (13).
Female infertility
The general principles outlined above may also apply to female infertile patients with raised oxidative stress. There are few studies on the role of antioxidants in female infertility (89, 90). Both the studies reported higher pregnancy rate with vitamin C supplementation compared to the control group. In vivo antioxidants may be helpful in infertile women who smoke, as history of smoking is associated with high levels of oxidative stress (55).
Use of antioxidants in IVF media appears to be useful in improving the pregnancy rates. Higher implantation and pregnancy rates were found when antioxidant supplemented media was used rather than standard media without antioxidants (91). A study from our center demonstrated that the adding antioxidants, especially vitamin C, can improve the blastocyst development rate in a mouse embryo model (92).
In ART procedures, sperm preparation techniques separate mature spermatozoa and thus minimize the interaction between ROS producing cells in semen (e.g. leukocytes, immature abnormal spermatozoa) and normal spermatozoa. Density gradient separation and swim-up methods are commonly used sperm preparation methods. Adding antioxidants to the sperm preparation media may help prevent ROS- induced damage and preserves the quality of spermatozoa during ART procedures.
SUMMARY
In conclusion, we feel that there is enough evidence in the literature to date to state that oxidants play an important role in human fertility. To answer the perennial question- whether oxidants are cause or effect of disease process, it appears that oxidants are the mediators of the final pathology and the cause of the clinical symptoms of the disease i.e., infertility in selected group of patients.
The physiological role of oxidants is gaining increasing attention with the publication of more studies on this topic. Oxidants appear to play a vital second messenger role in many cellular processes. Knowing the exact molecular mechanisms of their involvement in cell physiology may allow us to direct the development of new therapeutic drugs for infertility treatment.
The clinical estimation of oxidative stress and antioxidant supplementation in infertile men is being slowly recognized. However in case of women, research on role of oxidative stress is still in early stages. This may be due to the role of oxidants in multiple sites and the need for studies to address their role at each site separately (ovary, peritoneal cavity and uterus). Research is also hampered in females due to difficulty in accessing tissues for research, unlike the research on semen samples in men.
As of now there is no universally acceptable method of estimating oxidative stress. In view of the multiple variables that can affect the oxidative stress measurement, efforts should be focused to get a consensus on uniform protocols for oxidative stress estimation and expression. The normal clinical range of oxidative stress should be defined accurately. This is needed to document the increase in oxidative stress and thus to advise antioxidants based on evidence.
As production of oxidants is a ubiquitous phenomenon in every cell with oxidative phosphorylation/aerobic respiration, antioxidant systems appear to be very effective in human body. However, researchers have yet to identify key components of this system that can be targeted for therapeutic use. Presence of multiple antioxidant systems in the human body favors the use of antioxidants combination rather than single antioxidant supplementation. Studies looking in to the effectiveness of antioxidants in infertile patients are inconclusive, however in view of the available evidence, supplementation of antioxidants may be beneficial in selected patients with infertility. Randomized multi center trials are still needed to prove the effectiveness of the antioxidants in the treatment of infertility.
REFERENCES
- Agarwal A, Saleh RA. Role of oxidants in male infertility: rationale,
significance, and treatment. Urol Clin North Am 2002;29:817-27.
- Saleh RA, Agarwal A. Oxidative stress and male infertility: from research
bench to clinical practice. J Androl 2002;23:737-52.
- Agarwal A, Saleh RA, Bedaiwy MA. Role of reactive oxygen species in the
pathophysiology of human reproduction. Fertil Steril 2003;79:829-43.
- Halliwell B. Tell me about free radicals, doctor: a review. J R Soc Med 1989;82:747-52.
- Sharma RK, Agarwal A. Role of reactive oxygen species in male infertility.
Urology 1996;48:835-50.
- Sikka SC. Role of oxidative stress and antioxidants in andrology and assisted
reproductive technology. J Androl 2004;25:5-18.
- Gavella M, Lipovac V, Vucic M, Rocic B. Superoxide anion scavenging capacity
of human seminal plasma. Int J Androl 1996;19:82-90.
- Jozwik M, Kuczynski W, Szamatowicz M. Nonenzymatic antioxidant activity
of human seminal plasma. Fertil Steril 1997;68:154-7.
- Smith R, Vantman D, Ponce J, Escobar J, Lissi E. Total antioxidant capacity
of human seminal plasma. Hum Reprod 1996;11:1655-60.
- Zini A, Garrels K, Phang D. Antioxidant activity in the semen of fertile
and infertile men. Urology 2000;55:922-6.
- Jones R, Mann T, Sherins R. Peroxidative breakdown of phospholipids in
human spermatozoa, spermicidal properties of fatty acid peroxides, and protective
action of seminal plasma. Fertil Steril 1979;31:531-7.
- Sun JG, Jurisicova A, Casper RF. Detection of deoxyribonucleic acid fragmentation
in human sperm: correlation with fertilization in vitro. Biol Reprod 1997;56:602-7.
- Kodama H, Yamaguchi R, Fukuda J, Kasai H, Tanaka T. Increased oxidative
deoxyribonucleic acid damage in the spermatozoa of infertile male patients.
Fertil Steril 1997;68:519-24.
- Aitken RJ, Baker MA, Sawyer D. Oxidative stress in the male germ line and
its role in the aetiology of male infertility and genetic disease. Reprod
Biomed Online 2003;7:65-70.
- Duru N, Morshedi M, Oehninger S. Effects of hydrogen peroxide on DNA and
plasma membrane integrity of human spermatozoa. Fertil Steril 2000;74:1200
-7.
- Agarwal A, Said TM. Role of sperm chromatin abnormalities and DNA damage
in male infertility. Hum Reprod Update 2003;9:331-45.
- Twigg JP, Irvine DS, Aitken RJ. Oxidative damage to DNA in human spermatozoa
does not preclude pronucleus formation at intracytoplasmic sperm injection.
Hum Reprod 1998;13:1864-71.
- Marchetti C, Obert G, Deffosez A, Formstecher P, Marchetti P. Study of
mitochondrial membrane potential, reactive oxygen species, DNA fragmentation
and cell viability
by flow cytometry in human sperm. Hum Reprod 2002;17:1257-65.
- Sharma RK, Pasqualotto FF, Nelson DR, Thomas AJ, Jr., Agarwal A. The reactive
oxygen species-total antioxidant capacity score is a new measure of oxidative
stress to predict male infertility. Hum Reprod 1999;14:2801-7.
- MacLeod. The role of oxygen in the metabolism and motility of human spermatozoa.
Am J Physiol 1943;138:512.
- Aitken J, Fisher H. Reactive oxygen species generation and human spermatozoa:
the balance of benefit and risk. Bioessays 1994;16:259-67.
- Gil-Guzman E, Ollero M, Lopez MC, Sharma RK, Alvarez JG, Thomas AJ, Jr.,
et al. Differential production of reactive oxygen species by subsets of human
spermatozoa at different stages of maturation. Hum Reprod 2001;16:1922-30.
- Aitken RJ, Buckingham D, West K, Wu FC, Zikopoulos K, Richardson
DW. Differential contribution of leucocytes and spermatozoa to the generation
of reactive oxygen species in the ejaculates of oligozoospermic patients
and fertile donors. J Reprod Fertil 1992;94:451-62.
- Gavella M, Lipovac V. NADH-dependent oxidoreductase (diaphorase) activity
and isozyme pattern of sperm in infertile men. Arch Androl 1992;28:135-41.
- Alvarez JG, Touchstone JC, Blasco L, Storey BT. Spontaneous lipid peroxidation
and production of hydrogen peroxide and superoxide in human spermatozoa.
Superoxide dismutase as major enzyme protectant against oxygen toxicity.
J Androl 1987;8:338-48.
- Shekarriz M, Sharma RK, Thomas AJ, Jr., Agarwal A. Positive myeloperoxidase
staining (Endtz test) as an indicator of excessive reactive oxygen species
formation in semen. J Assist Reprod Genet 1995;12:70-4.
- Ochsendorf FR. Infections in the male genital tract and reactive oxygen
species. Hum Reprod Update 1999;5:399-420.
- Plante M, de Lamirande E, Gagnon C. Reactive oxygen species released by
activated neutrophils, but not by deficient spermatozoa, are sufficient to
affect normal sperm motility. Fertil Steril 1994;62:387-93.
- Aitken RJ, Gordon E, Harkiss D, Twigg JP, Milne P, Jennings Z, et al.
Relative impact of oxidative stress on the functional competence and genomic
integrity of human spermatozoa. Biol Reprod 1998;59:1037-46.
- Iwasaki A, Gagnon C. Formation of reactive oxygen species in spermatozoa
of infertile patients. Fertil Steril 1992;57:409-16.
- Armstrong JS, Rajasekaran M, Chamulitrat W, Gatti P, Hellstrom WJ, Sikka
SC. Characterization of reactive oxygen species induced effects on human
spermatozoa movement and energy metabolism. Free Radic Biol Med 1999;26:869-80.
- de Lamirande E, Gagnon C. Reactive oxygen species and human spermatozoa.
II. Depletion of adenosine triphosphate plays an important role in the inhibition
of sperm motility. J Androl 1992;13:379-86.
- Pasqualotto FF, Sharma RK, Nelson DR, Thomas AJ, Agarwal A. Relationship
between oxidative stress, semen characteristics, and clinical diagnosis in
men undergoing
infertility investigation. Fertil Steril 2000;73:459-64.
- Aitken RJ. The Amoroso Lecture. The human spermatozoon--a cell in
crisis? J Reprod Fertil 1999;115:1-7.
- Maiorino M, Ursini F. Oxidative stress, spermatogenesis and fertility.
Biol Chem 2002;383:591-7.
- Aitken RJ, Irvine DS, Wu FC. Prospective analysis of sperm-oocyte fusion
and reactive oxygen species generation as criteria for the diagnosis of infertility.
Am J Obstet Gynecol 1991;164:542-51.
- 37. Griveau JF, Le Lannou D. Reactive oxygen species
and human spermatozoa: physiology and pathology. Int J Androl 1997;20:61-9.
- Sakkas D, Mariethoz E, Manicardi G, Bizzaro D, Bianchi PG, Bianchi U. Origin
of DNA damage in ejaculated human spermatozoa. Rev Reprod 1999;4:31-7.
- Loft S, Kold-Jensen T, HjollundNH, Giwercman A, Gyllemborg J, Ernst E,
et al. Oxidative DNA damage in human sperm influences time to pregnancy.
Hum Reprod
2003;18:1265-72.
- Pasqualotto FF, Sharma RK, Potts JM, Nelson DR, Thomas AJ, Agarwal A. Seminal
oxidative stress in patients with chronic prostatitis. Urology 2000;55:881-5.
- Potts JM, Sharma R, Pasqualotto F, Nelson D, Hall G, Agarwal A. Association
of ureaplasma urealyticum with abnormal reactive oxygen species levels
and absence of leukocytospermia. J Urol 2000;163:1775-8.
- Vicari E. Effectiveness and limits of antimicrobial treatment on seminal
leukocyte concentration and related reactive oxygen species production in
patients with male accessory gland infection. Hum Reprod 2000;15:2536-44.
- de Lamirande E, Leduc BE, Iwasaki A, Hassouna M, Gagnon C. Increased reactive
oxygen species formation in semen of patients with spinal cord injury. Fertil
Steril 1995;63:637-42.
- Padron OF, Brackett NL, Sharma RK, Lynne CM, Thomas AJ, Jr., Agarwal A.
Seminal reactive oxygen species and sperm motility and morphology in men
with spinal
cord injury. Fertil Steril 1997;67:1115-20.
- Naughton CK, NangiaAK, Agarwal A. Pathophysiology of varicoceles in male
infertility. Hum Reprod Update 2001;7:473-81.
- Allamaneni SSR, Naughton CK, Sharma RK, Thomas AJ, Jr., Agarwal A. Increased
seminal reactive oxygen species levels in varicocele patients correlate with
varicocele grade, not testis size. Fertil Steril 2004 (In press).
- Hendin BN, Kolettis PN, Sharma RK, Thomas AJ, Jr., Agarwal A. Varicocele
is associated with elevated spermatozoal reactive oxygen species production
and diminished seminal plasma antioxidant capacity. J Urol 1999;161:1831-4.
- Barbieri ER, Hidalgo ME, Venegas A, Smith R, Lissi EA. Varicocele-associated
decrease in antioxidant defenses. J Androl 1999;20:713-7.
- Mostafa T, Anis TH, El-Nashar A, Imam H, OthmanIA. Varicocelectomy reduces
reactive oxygen species levels and increases antioxidant activity of seminal
plasma from infertile men with varicocele. Int J Androl 2001;24:261-5.
- Kolettis PN, Sharma RK, Pasqualotto FF, Nelson D, Thomas AJ, Jr., Agarwal
A. Effect of seminal oxidative stress on fertility after vasectomy reversal.
Fertil Steril 1999;71:249-55.
- Shapiro RH, Muller CH, Chen G, Berger RE. Vasectomy reversal associated
with increased reactive oxygen species production by seminal fluid leukocytes
and
sperm. J Urol 1998;160:1341-6.
- Saleh RA, Agarwal A, Sharma RK, Nelson DR, Thomas AJ, Jr. Effect of cigarette
smoking on levels of seminal oxidative stress in infertile men: a prospective
study. Fertil Steril 2002;78:491-9.
- Jozwik M, Wolczynski S, Szamatowicz M. Oxidative stress markers in preovulatory
follicular fluid in humans. Mol Hum Reprod 1999;5:409-13.
- Attaran M, Pasqualotto E, Falcone T, Goldberg JM, Miller KF, Agarwal A,
et al. The effect of follicular fluid reactive oxygen species on the outcome
of in vitro fertilization. Int J Fertil Womens Med 2000;45:314-20.
- Paszkowski T, Clarke RN, Hornstein MD. Smoking induces oxidative
stress inside the Graafian follicle. Hum Reprod 2002;17:921-5.
- Guerin P, El Mouatassim S, Menezo Y. Oxidative stress and protection against
reactive oxygen species in the pre-implantation embryo and its surroundings.
Hum Reprod Update 2001;7:175-89.
- Bedaiwy MA, Falcone T, Sharma RK, Goldberg JM, Attaran M, Nelson DR, et
al. Prediction of endometriosis with serum and peritoneal fluid markers:
a prospective controlled trial. Hum Reprod 2002;17:426-31.
- Riley JC, Behrman HR. Oxygen radicals and reactive oxygen species in reproduction.
Proc Soc Exp Biol Med 1991;198:781-91.
- Sugino N, Karube-Harada A, Taketani T, Sakata A, Nakamura Y. Withdrawal
of Ovarian Steroids Stimulates Prostaglandin F2alpha Production Through Nuclear
Factor-kappaB Activation via Oxygen Radicals in Human Endometrial Stromal
Cells:
Potential Relevance to Menstruation. J Reprod Dev 2004;50:215-25.
- Paszkowski T, Traub AI, Robinson SY, McMaster D. Selenium dependent glutathione
peroxidase activity in human follicular fluid. Clin Chim Acta 1995;236:173-80.
- Oyawoye O, Abdel Gadir A, Garner A, Constantinovici N, Perrett C, Hardiman
P. Antioxidants and reactive oxygen species in follicular fluid of women
undergoing IVF: relationship to outcome. Hum Reprod 2003;18:2270-4.
- Wang Y, Sharma RK, Falcone T, Goldberg J, Agarwal A. Importance of reactive
oxygen species in the peritoneal fluid of women with endometriosis or idiopathic
infertility. Fertil Steril 1997;68:826-30.
- Polak G, Koziol-Montewka M, Gogacz M, Blaszkowska I, Kotarski J. Total
antioxidant status of peritoneal fluid in infertile women. Eur J Obstet Gynecol
Reprod Biol 2001;94:261-3.
- Bedaiwy MA, Falcone T. Peritoneal fluid environment in endometriosis.
Clinicopathological implications. Minerva Ginecol 2003;55:333-45.
- Ota H, Igarashi S, Hatazawa J, Tanaka T. Endothelial nitric oxide synthase
in the endometrium during the menstrual cycle in patients with endometriosis
and adenomyosis. Fertil Steril 1998;69:303-8.
- Zeller JM, Henig I, Radwanska E, Dmowski WP. Enhancement of human monocyte
and peritoneal macrophage chemiluminescence activities in women with endometriosis.
Am J Reprod Immunol Microbiol 1987;13:78-82.
- Van Langendonckt A, Casanas-Roux F, Donnez J. Oxidative stress and peritoneal
endometriosis. Fertil Steril 2002;77:861-70.
- Murphy AA, Santanam N, Parthasarathy S. Endometriosis: a disease
of oxidative stress? Semin Reprod Endocrinol 1998;16:263-73.
- Strandell A, Lindhard A. Why does hydrosalpinx reduce fertility? The importance
of hydrosalpinx fluid. Hum Reprod 2002;17:1141-5.
- Bedaiwy MA, Goldberg JM, Falcone T, Singh M, Nelson D, Azab H, et al.
Relationship between oxidative stress and embryotoxicity of hydrosalpingeal
fluid. Hum Reprod 2002;17:601-4.
- Nasr-Esfahani MH, Winston NJ, Johnson MH. Effects of glucose, glutamine,
ethylenediaminetetraacetic acid and oxygen tension on the concentration of
reactive oxygen species and on development of the mouse preimplantation embryo
in vitro. J Reprod Fertil 1992;96:219-31.
- Goto Y, Noda Y, Mori T, Nakano M. Increased generation of reactive oxygen
species in embryos cultured in vitro. Free Radic Biol Med 1993;15:69-75.
- Yang HW, Hwang KJ, Kwon HC, Kim HS, Choi KW, Oh KS. Detection of reactive
oxygen species (ROS) and apoptosis in human fragmented embryos. Hum Reprod
1998;13:998-1002.
- Guyader-Joly C, Guerin P, Renard JP, Guillaud J, Ponchon S, Menezo Y.
Precursors of taurine in female genital tract: effects on developmental capacity
of bovine embryo produced in vitro. Amino Acids 1998;15:27-42.
- Paszkowski T, Clarke RN. The Graafian follicle is a site of L-ascorbate
accumulation. J Assist Reprod Genet 1999;16:41-5.
- Bedaiwy MA, Falcone T, Al-Hussaini T, Abdel-Aleem A, Sharma RK, Worley
SE, et al. Differential growth of human embryos in vitro: role of reactive
oxygen
species. Fertil Steril 2004;82:593-600
- Sukcharoen N, Keith J, Irvine DS, Aitken RJ. Predicting the fertilizing
potential of human sperm suspensions in vitro: importance of sperm morphology
and leukocyte
contamination. Fertil Steril 1995;63:1293-300.
- Zorn B, Vidmar G, Meden-Vrtovec H. Seminal reactive oxygen species as
predictors of fertilization, embryo quality and pregnancy rates after conventional
in vitro fertilization and intracytoplasmic sperm injection. Int J Androl
2003;26:279-85.
- Alvarez JG. Nurture vs nature: how can we optimize sperm quality? J Androl
2003;24:640-8.
- Agarwal A, Nallella KP, Allamaneni SSR, Said TM. Role of antioxidants
in treatment of male infertility: an overview of the literature. Reprod Biomed
Online 2004;8:616-27.
- Suleiman SA, Ali ME, Zaki ZM, el-Malik EM, Nasr MA. Lipid peroxidation
and human sperm motility: protective role of vitamin E. J Androl 1996;17:530-7.
- Vezina D, Mauffette F, Roberts KD, Bleau G. Selenium-vitamin E supplementation
in infertile men. Effects on semen parameters and micronutrient levels
and distribution. Biol Trace Elem Res 1996;53:65-83.
- Lenzi A, Picardo M, Gandini L, Lombardo F, Terminali O, Passi S, et al.
Glutathione treatment of dyspermia: effect on the lipoperoxidation process.
Hum Reprod
1994;9:2044-50.
- Comhaire FH, Christophe AB, Zalata AA, Dhooge WS, Mahmoud AM, Depuydt
CE. The effects of combined conventional treatment, oral antioxidants and
essential fatty acids on sperm biology in subfertile men. Prostaglandins
Leukot Essent Fatty Acids 2000;63:159-65.
- Vicari E, Calogero AE. Effects of treatment with carnitines in infertile
patients with prostato-vesiculo-epididymitis. Hum Reprod 2001;16:2338-42.
- Vicari E, La Vignera S, Calogero AE. Antioxidant treatment with carnitines
is effective in infertile patients with prostatovesiculoepididymitis and
elevated seminal leukocyte concentrations after treatment with nonsteroidal
anti-inflammatory compounds. Fertil Steril 2002;78:1203-8.
- Lenzi A, Lombardo F, Sgro P, Salacone P, Caponecchia L, Dondero F, et
al. Use of carnitine therapy in selected cases of male factor infertility:
a double-blind crossover trial. Fertil Steril 2003;79:292-300.
- Lewin A, Lavon H. The effect of coenzyme Q10 on sperm motility and function.
Mol Aspects Med 1997;18 Suppl:S213-9.
- Henmi H, Endo T, Kitajima Y, Manase K, Hata H, Kudo R. Effects of ascorbic
acid supplementation on serum progesterone levels in patients with a luteal
phase defect. Fertil Steril 2003;80:459-61.
- Crha I, Hruba D, Ventruba P, Fiala J, Totusek J, Visnova H. Ascorbic acid
and infertility treatment. Cent Eur J Public Health 2003;11:63-7.
- Catt JW, Henman M. Toxic effects of oxygen on human embryo development.
Hum Reprod 2000;15 Suppl 2:199-206.
- Wang X, Falcone T, Attaran M, Goldberg JM, Agarwal A, Sharma RK. Vitamin
C and vitamin E supplementation reduce oxidative stress-induced embryo toxicity
and improve the blastocyst development rate. Fertil Steril 2002;78:1272-7.
Copyright © Middle East Fertility Society
Untitled Document
Middle East Fertility Society Journal, Vol. 9, No. 3, 2004, pp.
187-197
REVIEW
Oxidants and antioxidants in human fertility
Ashok Agarwal, Ph.D., HCLD., Shyam S.R. Allamaneni, M.D.
Center for Advanced Research in Human
Reproduction, Infertility and Sexual Function, Glickman Urological Institute
and Department of Obstetrics and Gynecology, Cleveland Clinic Foundation,
Cleveland, Ohio.
Correspondence and reprint requests: Dr. Ashok Agarwal, Director, Center for Advanced Research in Human, Reproduction, Infertility, and Sexual Function, Glickman Urological Institute and Department of Obstetrics-Gynecology, The Cleveland Clinic Foundation, 9500 Euclid Avenue, Desk A19.1, Cleveland, OH 44195, USA (FAX: 216-445-6049; e-mail: agarwaa@ccf.org).
Received on June 15, 2004;
revised and accepted on August 29, 2004
Code Number: mf04034
ABSTRACT
Oxidants are highly unstable molecules
that attack every chemical substance they come into contact. Oxidants modify
the macromolecules both structurally and functionally. Body has defense
mechanisms against oxidants in the form of both enzymatic and non-enzymatic
antioxidants. Reactive oxygen species (ROS) are a group of oxidants formed
during oxygen metabolism. ROS appears to be involved in the pathogenesis of
many human diseases. In reproductive medicine, ROS have both physiological and
pathological role in male and female reproduction. Oxidative stress develops
when the generation of ROS overwhelms the scavenging capacity of antioxidants.
Oxidative stress causes damage to spermatozoa, oocyte and embryos. It appears
to play a role in both natural and in vitro fertilization and pregnancy. The
patients with oxidative stress may benefit from the strategies to reduce
oxidative stress and treatment with antioxidants.
Key words: Oxidants, antioxidants, infertility - male & female, spermatozoa, reactive
oxygen species, free radicals.
INTRODUCTION
Infertility can be defined as a lack of pregnancy after one year of regular unprotected intercourse. Approximately 15%-20% of couples of reproductive age are infertile, which can be attributed equally to both male and female factors. Recent research on the role of reactive oxygen species (ROS) in human infertility has received a great deal of interest from the scientists and medical practitioners (1-3). This review summarizes the latest consensus on the role of ROS in human reproduction. It should help clarify the role of ROS in human fertility and may lead into the development of newer therapeutic approaches to treat infertility.
Oxidants
Reactive oxygen species (ROS) are oxygen-derived molecules, which are formed as intermediary products and are a class of powerful oxidants in the human body. ROS include superoxide anion (O2((), hydrogen peroxide (H2O2) and hydroxyl radical (OH.). Some cells posses specific mechanisms to produce ROS that are required for cellular functions in low concentrations (4). Aerobic environment is a constant source of ROS through in vivo mechanisms such as electron leakage during biologic oxidations, and by physical activation of oxygen by external agents such as irradiation, e.g. UV sunlight. ROS are characterized by their ability to react with any molecule they come in contact and modify it oxidatively. The modification may result in structural and functional alterations and impair many cellular processes. Depending on their tissue concentration they can either exert beneficial physiologic effects (e.g. play role in fertilization process) or pathological damage to cellular components, including lipids, proteins and nucleic acids (5).
Antioxidants
Organisms have developed efficient protective mechanisms against excessive accumulation of ROS. ROS are neutralized by an elaborate antioxidant defense system consisting of enzymes such as catalase, superoxide dismutase and glutathione peroxidase/reductase, and numerous non-enzymatic antioxidants such as vitamin C, vitamin E, vitamin A, pyruvate, glutathione, taurine and hypotaurine (6). In a healthy body, pro-oxidants and antioxidants maintain a ratio and a shift in this ratio towards pro-oxidants gives rise to oxidative stress. This oxidative stress may be either mild or severe depending on the extent of shift. Whenever ROS levels become pathologically elevated, antioxidants begin to work and help minimize the oxidative damage, repair it or prevent it altogether. The male and female genital tracts are rich in both enzymatic and non-enzymatic antioxidants (7-10). Vitamins C and E act as chain-breaking antioxidants and thus prevent the propagation of peroxidative process.
OXIDANTS AND PATHOLOGICAL MECHANISMS OF CELL INJURY
Lipid peroxidation
ROS can attack polyunsaturated fatty acids in the cell membrane leading to a chain of chemical reactions called lipid peroxidation. Fatty acid breakdown results in the formation of various oxidatively modified products, which are toxic to cells and are finally converted into stable end products. The spermatozoal membrane contains large amounts of polyunsaturated fatty acids (11), which maintain its fluidity. Peroxidation of these fatty acids leads to the loss of membrane fluidity and a reduction in the activity of membrane enzymes and ion channels. As a result, the normal cellular mechanisms that are required for fertilization are inhibited. It is possible to measure the extent of peroxidative damage by estimating the stable end products of lipid peroxidation such as malondialdehyde (5).
DNA damage
Susceptibility of DNA to oxidative damage is indicated by the presence of oxidatively modified substances like 8-hydroxy-2-deoxyguanosine. Deoxyribonucleic acid bases and phosphodiester backbones are sites that are susceptible to peroxidative damage. High levels of ROS mediate the DNA fragmentation that is commonly observed in the spermatozoa of infertile men (12, 13). Normally, sperm DNA is protected from oxidative insult by its specific compact organization and by antioxidants in the seminal plasma. Spermatozoa are unique in that they cannot repair DNA and depend on the oocyte for repair after fertilization (14). Various types of DNA abnormalities occur in sperm that have been exposed to ROS artificially. These abnormalities include base modification, production of base-free sites, deletions, frame shifts, DNA cross-links and chromosomal rearrangements (15, 16). Patients with high levels of oxidative stress in their seminal fluid were found to have sperm with multiple single and double DNA strand breaks (17). A biomarker for oxidative DNA damage, 8-hydroxy-2-deoxyguanosine, can be used to determine the extent of ROS-induced DNA damage.
Apoptosis
ROS may also initiate a chain of reactions that ultimately lead to apoptosis.
Apoptosis is a natural process in which the body removes old and senescent
cells; it is a process of programmed cell death. In human germ cells, apoptosis
may help remove abnormal germ cells and prevent their overproduction. Multiple
extrinsic and intrinsic cell factors control the process of apoptosis (3).
In a study from our center, levels of ROS were positively associated with apoptosis
in mature spermatozoa. Levels of caspases, which are proteases involved in
apoptosis, correlated with levels of ROS. Our results also showed that apoptosis
could be induced in cell cultures with H2O2, which further supports the theory
that ROS is involved in apoptosis. The process of apoptosis may also be accelerated
by ROS-induced DNA damage and ultimately may lead to a decline in sperm count
(6).
OXIDATIVE STRESS MEASUREMENT
Oxidative stress can be estimated directly or indirectly. The direct measurement of ROS is by using electron spin resonance method and is used sparingly in reproductive medicine. Indirect tests measure oxidatively modified products. Chemiluminescence is a common method used and is based on emission of light on chemiluminescent reaction between ROS and reagent (luminal/lucigenin). The amount of light emitted is quantified and measured by a luminometer.
Lipid peroxidation end products like malondialdehyde, lipid hydroperoxides, and conjugated dienes are commonly used to assess the oxidative stress. Other methods are measurement of protein and DNA oxidation products, and changes in status of antioxidants. Flow cytometry is also being used to measure the individual ROS radicals (18).
ROS-TAC score
It is a concept proposed by our group to represent the oxidative stress status of individual more accurately. This score accommodate for the variations in both ROS and TAC (total antioxidant capacity) values (19). Fertile men tend to have high ROS-TAC scores whereas infertile men generally have significantly lower scores. ROS levels can also be measured directly in neat semen, thereby offering yet another measure of oxidative stress.
Oxidative Stress and Male Infertility
The presence of free radicals in the spermatozoa was reported by MacLeod 50 years ago (20). Because spermatozoa lack cytoplasmic enzymes, they often are unable to prevent oxidative damage by these free radicals. This is one of the features that make spermatozoa highly susceptible to peroxidative damage. Most cytoplasmic enzymes are extruded during the final stages of the sperm maturation process, which enables sperm to attain their characteristic morphology (21). Nature compensated for this deficiency by providing an array of antioxidants in the seminal plasma.
Sources of oxidants
Morphologically abnormal spermatozoa and leukocytes are the major sources of ROS in the male reproductive tract. Even though mature spermatozoa may not produce pathologically significant levels of ROS, oxidative damage may occur in the epididymis and seminiferous tubules where they are in close contact with the immature, ROS producing spermatozoa and leukocytes (22).
ROS production is elevated in patients who have a large percentage of spermatozoa with excess residual cytoplasm in the midpiece (22). Excess residual cytoplasm contains enzymes such as glucose-6-phosphate dehydrogenase and creatine phosphokinase, which are linked with generation of ROS and defective sperm function. ROS may be generated at the level of plasma membrane (NADPH-oxidase system) (23) or mitochondria (NADH-dependent oxido-reductase) (24). Human spermatozoa generate O2(( (25), which spontaneously or enzymatically dismutates to H2O2. In the presence of metal ions (iron)- O2(( and H2O2 together produces the more harmful oxidant, OH..
Neutrophils and macrophages are the major source of oxidants in the reproductive tract (26, 27). During inflammation and infection, activated leukocytes can produce significantly higher amounts of ROS than non-activated leukocytes (28). The ROS production in leukocytes is through NADPH oxidase enzyme. Even though ROS is released as part of defense mechanism in to the reproductive tract, it can damage surrounding spermatozoa, especially when antioxidant systems are overwhelmed. The importance of leukocyte contamination in producing ROS is well observed in Percoll-washed spermatozoa where a small number of leukocytes produce ROS. Increased levels of seminal leukocytes may also stimulate human spermatozoa to produce ROS. Such stimulation may be mediated via direct cell-cell contact or by soluble products released by leukocytes (27).
Mechanism of loss of sperm function
The mechanism of loss of sperm function by ROS appears to be multifold (29). ROS may affect the quality and number of spermatozoa reaching the ovum in the female reproductive tract. In addition, ROS impair the fertilization process by preventing the initiation of sperm-oocyte fusion events (14). Finally, ROS can impair embryo development and affect the health of offspring by damaging sperm DNA (16).
Impairment of standard semen parameters
Motility is a very important attribute unique to spermatozoa in entire human cells. Motility is indispensable to the spermatozoa, as it has to travel the female reproductive tract to reach the site of fertilization. Studies found that levels of ROS correlate with motility of spermatozoa (30, 31). In vitro studies showed that the impaired motility may be a temporary event or permanent phenomena. Excessive ROS causes ATP to deplete rapidly resulting in decreased phosphorylation of axonemal proteins and cause transient impairment of motility (32). Peroxidative damage to the sperm membrane and axonemal proteins appears to be the cause of permanent impairment in sperm motility.
ROS appears to play a role in the apoptosis of spermatozoa by activating caspases. Under normal conditions, abnormal sperm undergo apoptosis, which minimizes their presence in the semen. The severity of oligozoospermia has been correlated with excessive levels of ROS (33). ROS may stimulate the process of apoptosis, resulting in the death of spermatozoa and decreased sperm count (6). Patients with a low sperm count have a reduced chance of initiating a pregnancy. In view of recent reports of declining sperm counts during the last several decades, a possible association between oxidative damage to spermatozoa and declining sperm count in the general population is postulated (34).
Impairment of sperm-oocyte fusion
A minimal amount of ROS is required for the normal sperm-oocyte fusion. Spermatozoa and oocyte has inbuilt mechanism to prevent excessive production of ROS at the time of sperm-oocyte fusion, this may be by the release of SOD (superoxide dismutase) (35). If there is an abnormality in the production of SOD, ROS generation can continue uninterruptedly and damage both spermatozoa and oocyte. The affect of ROS on sperm fertilizing capacity cannot be quantified by measuring routine semen parameters. It is possible that the levels of ROS needed to impair sperm-oocyte fusion events are lower than those required to affect sperm motility. The inability of sperm to fuse with an oocyte appears to be due to the effects of ROS on the sperm membrane. The lipid peroxidation process results in loss of membrane fluidity due to disorganization of membrane architecture and reduction in the activity of membrane enzymes and ion channels. As a result, spermatozoa are unable to initiate the necessary biochemical reactions associated with acrosome reaction, zona pellucida binding and oocyte penetration (36, 37).
Sperm DNA damage
Sperm DNA contributes the half of genomic material to the offspring. Thus, normal sperm genetic material is required for fertilization, embryo and fetus development and postnatal child well being (16, 38). A recent study showed decreasing likelihood of pregnancy with increasing levels of 8-hydroxy-2-deoxyguanosine, an indicator of oxidative damage to DNA (39). The percentage of sperm with DNA damage is negatively correlated with the fertilization rate (12). Oocytes can repair DNA damage to some extent, but when the damage is severe, embryo death and miscarriages can occur. The affect of ROS on DNA integrity has become the focus of recent attention due to widespread use of assisted reproduction techniques (ART) such as intracytoplasmic injection (ICSI). In natural pregnancy, oxidative damage to the sperm membrane may ensure that spermatozoa with damaged DNA lose their ability to fertilize an oocyte. However, sperm with DNA damage can potentially be injected into an oocyte in the ICSI resulting in fertilization and pregnancy which may progress to live birth with congenital abnormalities (34).
Male infertility, oxidative stress and clinical applications
For the clinician it is important to know the clinical conditions in which oxidative stress may play a role in the etiology of infertility. Many clinical conditions were found to be associated with increased oxidative stress (33). Infections and inflammations involving the male reproductive tract are obvious conditions associated with oxidative stress in view of excessive generation of ROS by leukocytes (40-42). Very high percentage of spinal cord injury patients were reported to have elevated levels of oxidative stress (43, 44). Mechanism of infertility in patients with varicocele is poorly understood and ROS is postulated as a possible mediator (45, 46). Elevated levels of ROS and depressed levels of TAC were associated with varicocele (47-49). Patients who underwent vasectomy reversal also had high levels of reactive oxygen species (50, 51). A history of smoking was associated with high levels of oxidative stress (52).
ROLE OF OXIDANTS IN FEMALE INFERTILITY
The role of oxidative stress in female reproductive diseases and infertility is under intense investigations. Many studies reported the presence of oxidative and antioxidant systems in various female reproductive tissues (53-57). ROS appears to have physiological role in female reproductive tract in many different processes such as: oocyte maturation, fertilization, luteal regression, and endometrial shedding (58, 59). ROS levels in follicular fluid may be used as markers for predicting the success of in vitro fertilization (IVF)(3).
Whenever there is imbalance in the levels of ROS and antioxidants- damage can occur to oocytes and embryos through various pathological mechanisms described previously. Oxidative stress can affect the female fertility potential in number of ways. It may affect the ovulation, fertilization, embryo development and implantation.
The sources of ROS in Graffian follicle may be macrophages, neutrophils and granulosa cells. Follicular fluid contains high levels of antioxidants, which protect oocytes from ROS-induced damage. Significantly lower selenium levels were detected in follicular fluid of patients with unexplained infertility compared with those with tubal infertility or couples with male factor infertility (60). Another study reported that baseline TAC levels were higher in follicles whose oocytes fertilized successfully (61).
Elevated levels of ROS in peritoneal fluid may be the cause of infertility in some women who do not have any other obvious cause. Elevated levels can damage the ovum after its release from the ovary, the zygote/embryo and most importantly, spermatozoa. As discussed previously, spermatozoa are very sensitive to oxidative stress. Studies have compared ROS levels in peritoneal fluid between women undergoing laparoscopy for infertility evaluation and fertile women undergoing tubal ligation. ROS levels in the peritoneal fluid were significantly higher in the patients with idiopathic infertility compared with the fertile women (57, 62). High levels of malondialdehyde and low levels of antioxidants in the peritoneal fluid were reported in patients with unexplained infertility compared to controls (63).
Do oxidants have a role in pathogenesis of the endometriosis?
Oxidative stress is postulated as one of the possible mechanism of endometriosis. (64). The endometrial tissue has multiple cells like macrophages, red blood cells, which can generate ROS. Studies of women with endometriosis have suggested that peritoneal macrophages are responsible for increased production of ROS or increased expression of xanthine oxidase in endometrial cells (65, 66). High levels of oxidatively modified substances in peritoneal fluid and ectopic endometrial tissue were reported (67). Altered expression of defensive antioxidant enzymes and low levels of vitamin E were reported in patients with endometriosis (68). However, ROS levels showed no difference between healthy women and those with endometriosis (57, 62). Thus, more studies are needed to clearly understand the role of ROS in endometriosis.
Oxidants and hydrosalpingeal fluid
Hydrosalpinx has been associated with decreased outcome after IVF. But, the toxic agent associated with hydrosalpinx is not clearly known (69). A study from our center demonstrated the presence of ROS, antioxidants and lipid peroxidation products in hydrosalpingeal fluid (HSF) (70). We found that mouse embryo blastocyst development rate was higher when they were incubated with HSF containing low ROS levels than when incubated with HSF without ROS. These low ROS levels may in fact represent the physiological levels and thus account for observed positive relationship between ROS levels and blastocyst development rate.
Effect of Oxidants on Embryo Growth
Oxidative stress appears to have a detrimental effect on the development of embryo. ROS may originate from embryo metabolism and from the surrounding environment (71, 72). ROS not only alters most types of cellular molecules but also induces early embryonic developmental block and retardation (56). High levels of ROS and apoptosis were reported in fragmented embryos compared to non-fragmented embryos (73). Multiple mechanisms of embryo protection against ROS exist (74, 75).
According to a study from our center, ROS levels in day 1 culture media can help predict the success of fertilization, embryo development and pregnancy (76). In our study, ROS levels in Day 1 culture media correlated well with fertilization and embryo development in patients undergoing IVF and ICSI. They also correlated with pregnancy in ICSI but not in IVF.
Effects of Oxidants on In Vitro Reproduction
DNA damage induced by oxidative stress has important clinical implications in the context of assisted reproduction. Spermatozoa selected for ART most likely originate from an environment experiencing OS, and a large percentage of these sperm may have damaged DNA (2). There is a strong possibility that spermatozoa with damaged DNA may be used during ART (16), which can negatively affect the ART success rate and increase the risk of spontaneous abortion or offspring with genetic disorders. ROS levels in mature spermatozoa correlate significantly with the fertilizing potential of spermatozoa (77, 78). Estimating ROS levels may help predict the success rate of assisted reproduction procedures.
ROS and Sperm Preparation
A possible source of ROS in ART media is during the preparation of semen. Sperm preparation is necessary to enhance and maintain sperm quality and function after ejaculation before the semen specimen can be used for ART procedures (79). The production of ROS may be due to either 1) activation of spermatozoa by centrifugation process, 2) absence of antioxidant rich seminal plasma, or 3) because of minimal contamination of ROS produced by leukocytes and abnormal spermatozoa. The small amount of ROS produced may not decrease motility but can still cause DNA damage (29). A proper sperm preparation method should be selected so as to decrease the production of ROS.
THERAPEUTIC INTERVENTION AGAINST OXIDANTS
General treatment strategies
In both male and female reproduction, oxidative stress appears to be due to increased generation of ROS rather than a depletion of antioxidants. It is important to identify the source of increased ROS generation (80). The underlying etiological factor for abnormal leukocyte infiltration (e.g. leukocytospermia, inflammation, infection, smoking) should be determined. Patients with history of smoking should be advised to stop smoking. Any exposure to drugs, toxic substances and radiation should be checked and patients advised to stop exposure to them. Infections of the reproductive tract should be treated with appropriate antibiotics.
Initially, specific therapeutic options directed against the etiological cause of raised ROS should be tried. Patients with reproductive tract infection should be treated with antibiotics. Anti-inflammatory agents may help patients with persistent leukocytospermia and elevated levels of cytokines. Antioxidant supplementation may or may not be effective depending on the pathology of the infertility. After treating the primary cause (such as varicocele), patients can be advised to take antioxidant supplementation. Antioxidants can be started directly when a specific etiology cannot be identified (idiopathic infertility).
Male infertility
Semen analysis should be repeated after a full spermatogenic cycle in those men showing large number of abnormal spermatozoa with excessive cytoplasm in the mid piece during a routine analysis. This can help distinguish between a temporary disturbance in spermatogenesis and a permanent defect in spermatogenesis. Varicocelectomy may remove an unknown stimulus of ROS generation. Even though there is no definitive consensus on the use of antioxidants, many in vitro and in vivo studies have shown that they improve semen quality and fertility (80).
Some studies showed improvement in terms of pregnancy rate after antioxidants supplementation. Oral vitamin E is an antioxidant favored by many researchers and clinicians. Many formulations of vitamin E are available as oral supplements. Oral administration of 300 mg twice a day of vitamin E in a randomized double blind placebo controlled trial showed significant improvement of pregnancy rates (21%; 11/52) in infertile (asthenozoospermic) patients, while resulting in lack of pregnancies in the placebo group. This study also found significant improvement in sperm motility, and reduced lipid peroxidation levels after vitamin E supplementation (81). A combination of vitamin E and selenium in oligoasthenoteratozoospermic (OAT) patients resulted in significant improvement in sperm motility, viability and morphology (82).
Treatment may be more appropriate if antioxidants are given to the patients with raised ROS levels. In one such study, when infertile patients with high ROS production were treated with GSH (reduced glutathione) (600 mg), a positive effect on sperm motility, on sperm morphology and significantly reduced lipid peroxidation levels were observed after 30 days of treatment (83).
The efficacy of antioxidants may be evaluated by measuring ROS levels during treatment. But, from a clinical point of view, improved pregnancy rate may be a more relevant end point. The combination therapy of vitamin E with N-acetyl-cysteine (NAC) or vitamins A plus E and essential fatty acids significantly reduced ROS and improved pregnancy rates (84). Several studies have demonstrated the ability of carnitines (L-carnitine 1 g and acetyl-carnitine 0.5 g twice a day) to significantly reduce the ROS production and increase the chances of a couple to become pregnant (85-87).
The importance of studying sperm function tests or pregnancy rate is emphasized by observation that the improvement in the fertilization rate is not always accompanied by a concomitant improvement in sperm parameters. Treatment of subfertile patients with low fertilization rates after ICSI with oral coenzyme Q10 60 mg/day for 103 days, improved fertilization rate in ICSI, although no significant improvement was observed in sperm parameters (88).
Some studies reported antioxidants effect in terms of their ability to decrease sperm DNA damage levels. Oral administration of 200 mg of vitamin C, 200 mg of vitamin E and 400 mg of GSH for 2 months significantly improved serum levels of antioxidants and relatively decreased sperm DNA damage (13).
Female infertility
The general principles outlined above may also apply to female infertile patients with raised oxidative stress. There are few studies on the role of antioxidants in female infertility (89, 90). Both the studies reported higher pregnancy rate with vitamin C supplementation compared to the control group. In vivo antioxidants may be helpful in infertile women who smoke, as history of smoking is associated with high levels of oxidative stress (55).
Use of antioxidants in IVF media appears to be useful in improving the pregnancy rates. Higher implantation and pregnancy rates were found when antioxidant supplemented media was used rather than standard media without antioxidants (91). A study from our center demonstrated that the adding antioxidants, especially vitamin C, can improve the blastocyst development rate in a mouse embryo model (92).
In ART procedures, sperm preparation techniques separate mature spermatozoa and thus minimize the interaction between ROS producing cells in semen (e.g. leukocytes, immature abnormal spermatozoa) and normal spermatozoa. Density gradient separation and swim-up methods are commonly used sperm preparation methods. Adding antioxidants to the sperm preparation media may help prevent ROS- induced damage and preserves the quality of spermatozoa during ART procedures.
SUMMARY
In conclusion, we feel that there is enough evidence in the literature to date to state that oxidants play an important role in human fertility. To answer the perennial question- whether oxidants are cause or effect of disease process, it appears that oxidants are the mediators of the final pathology and the cause of the clinical symptoms of the disease i.e., infertility in selected group of patients.
The physiological role of oxidants is gaining increasing attention with the publication of more studies on this topic. Oxidants appear to play a vital second messenger role in many cellular processes. Knowing the exact molecular mechanisms of their involvement in cell physiology may allow us to direct the development of new therapeutic drugs for infertility treatment.
The clinical estimation of oxidative stress and antioxidant supplementation in infertile men is being slowly recognized. However in case of women, research on role of oxidative stress is still in early stages. This may be due to the role of oxidants in multiple sites and the need for studies to address their role at each site separately (ovary, peritoneal cavity and uterus). Research is also hampered in females due to difficulty in accessing tissues for research, unlike the research on semen samples in men.
As of now there is no universally acceptable method of estimating oxidative stress. In view of the multiple variables that can affect the oxidative stress measurement, efforts should be focused to get a consensus on uniform protocols for oxidative stress estimation and expression. The normal clinical range of oxidative stress should be defined accurately. This is needed to document the increase in oxidative stress and thus to advise antioxidants based on evidence.
As production of oxidants is a ubiquitous phenomenon in every cell with oxidative phosphorylation/aerobic respiration, antioxidant systems appear to be very effective in human body. However, researchers have yet to identify key components of this system that can be targeted for therapeutic use. Presence of multiple antioxidant systems in the human body favors the use of antioxidants combination rather than single antioxidant supplementation. Studies looking in to the effectiveness of antioxidants in infertile patients are inconclusive, however in view of the available evidence, supplementation of antioxidants may be beneficial in selected patients with infertility. Randomized multi center trials are still needed to prove the effectiveness of the antioxidants in the treatment of infertility.
REFERENCES
- Agarwal A, Saleh RA. Role of oxidants in male infertility: rationale,
significance, and treatment. Urol Clin North Am 2002;29:817-27.
- Saleh RA, Agarwal A. Oxidative stress and male infertility: from research
bench to clinical practice. J Androl 2002;23:737-52.
- Agarwal A, Saleh RA, Bedaiwy MA. Role of reactive oxygen species in the
pathophysiology of human reproduction. Fertil Steril 2003;79:829-43.
- Halliwell B. Tell me about free radicals, doctor: a review. J R Soc Med 1989;82:747-52.
- Sharma RK, Agarwal A. Role of reactive oxygen species in male infertility.
Urology 1996;48:835-50.
- Sikka SC. Role of oxidative stress and antioxidants in andrology and assisted
reproductive technology. J Androl 2004;25:5-18.
- Gavella M, Lipovac V, Vucic M, Rocic B. Superoxide anion scavenging capacity
of human seminal plasma. Int J Androl 1996;19:82-90.
- Jozwik M, Kuczynski W, Szamatowicz M. Nonenzymatic antioxidant activity
of human seminal plasma. Fertil Steril 1997;68:154-7.
- Smith R, Vantman D, Ponce J, Escobar J, Lissi E. Total antioxidant capacity
of human seminal plasma. Hum Reprod 1996;11:1655-60.
- Zini A, Garrels K, Phang D. Antioxidant activity in the semen of fertile
and infertile men. Urology 2000;55:922-6.
- Jones R, Mann T, Sherins R. Peroxidative breakdown of phospholipids in
human spermatozoa, spermicidal properties of fatty acid peroxides, and protective
action of seminal plasma. Fertil Steril 1979;31:531-7.
- Sun JG, Jurisicova A, Casper RF. Detection of deoxyribonucleic acid fragmentation
in human sperm: correlation with fertilization in vitro. Biol Reprod 1997;56:602-7.
- Kodama H, Yamaguchi R, Fukuda J, Kasai H, Tanaka T. Increased oxidative
deoxyribonucleic acid damage in the spermatozoa of infertile male patients.
Fertil Steril 1997;68:519-24.
- Aitken RJ, Baker MA, Sawyer D. Oxidative stress in the male germ line and
its role in the aetiology of male infertility and genetic disease. Reprod
Biomed Online 2003;7:65-70.
- Duru N, Morshedi M, Oehninger S. Effects of hydrogen peroxide on DNA and
plasma membrane integrity of human spermatozoa. Fertil Steril 2000;74:1200
-7.
- Agarwal A, Said TM. Role of sperm chromatin abnormalities and DNA damage
in male infertility. Hum Reprod Update 2003;9:331-45.
- Twigg JP, Irvine DS, Aitken RJ. Oxidative damage to DNA in human spermatozoa
does not preclude pronucleus formation at intracytoplasmic sperm injection.
Hum Reprod 1998;13:1864-71.
- Marchetti C, Obert G, Deffosez A, Formstecher P, Marchetti P. Study of
mitochondrial membrane potential, reactive oxygen species, DNA fragmentation
and cell viability
by flow cytometry in human sperm. Hum Reprod 2002;17:1257-65.
- Sharma RK, Pasqualotto FF, Nelson DR, Thomas AJ, Jr., Agarwal A. The reactive
oxygen species-total antioxidant capacity score is a new measure of oxidative
stress to predict male infertility. Hum Reprod 1999;14:2801-7.
- MacLeod. The role of oxygen in the metabolism and motility of human spermatozoa.
Am J Physiol 1943;138:512.
- Aitken J, Fisher H. Reactive oxygen species generation and human spermatozoa:
the balance of benefit and risk. Bioessays 1994;16:259-67.
- Gil-Guzman E, Ollero M, Lopez MC, Sharma RK, Alvarez JG, Thomas AJ, Jr.,
et al. Differential production of reactive oxygen species by subsets of human
spermatozoa at different stages of maturation. Hum Reprod 2001;16:1922-30.
- Aitken RJ, Buckingham D, West K, Wu FC, Zikopoulos K, Richardson
DW. Differential contribution of leucocytes and spermatozoa to the generation
of reactive oxygen species in the ejaculates of oligozoospermic patients
and fertile donors. J Reprod Fertil 1992;94:451-62.
- Gavella M, Lipovac V. NADH-dependent oxidoreductase (diaphorase) activity
and isozyme pattern of sperm in infertile men. Arch Androl 1992;28:135-41.
- Alvarez JG, Touchstone JC, Blasco L, Storey BT. Spontaneous lipid peroxidation
and production of hydrogen peroxide and superoxide in human spermatozoa.
Superoxide dismutase as major enzyme protectant against oxygen toxicity.
J Androl 1987;8:338-48.
- Shekarriz M, Sharma RK, Thomas AJ, Jr., Agarwal A. Positive myeloperoxidase
staining (Endtz test) as an indicator of excessive reactive oxygen species
formation in semen. J Assist Reprod Genet 1995;12:70-4.
- Ochsendorf FR. Infections in the male genital tract and reactive oxygen
species. Hum Reprod Update 1999;5:399-420.
- Plante M, de Lamirande E, Gagnon C. Reactive oxygen species released by
activated neutrophils, but not by deficient spermatozoa, are sufficient to
affect normal sperm motility. Fertil Steril 1994;62:387-93.
- Aitken RJ, Gordon E, Harkiss D, Twigg JP, Milne P, Jennings Z, et al.
Relative impact of oxidative stress on the functional competence and genomic
integrity of human spermatozoa. Biol Reprod 1998;59:1037-46.
- Iwasaki A, Gagnon C. Formation of reactive oxygen species in spermatozoa
of infertile patients. Fertil Steril 1992;57:409-16.
- Armstrong JS, Rajasekaran M, Chamulitrat W, Gatti P, Hellstrom WJ, Sikka
SC. Characterization of reactive oxygen species induced effects on human
spermatozoa movement and energy metabolism. Free Radic Biol Med 1999;26:869-80.
- de Lamirande E, Gagnon C. Reactive oxygen species and human spermatozoa.
II. Depletion of adenosine triphosphate plays an important role in the inhibition
of sperm motility. J Androl 1992;13:379-86.
- Pasqualotto FF, Sharma RK, Nelson DR, Thomas AJ, Agarwal A. Relationship
between oxidative stress, semen characteristics, and clinical diagnosis in
men undergoing
infertility investigation. Fertil Steril 2000;73:459-64.
- Aitken RJ. The Amoroso Lecture. The human spermatozoon--a cell in
crisis? J Reprod Fertil 1999;115:1-7.
- Maiorino M, Ursini F. Oxidative stress, spermatogenesis and fertility.
Biol Chem 2002;383:591-7.
- Aitken RJ, Irvine DS, Wu FC. Prospective analysis of sperm-oocyte fusion
and reactive oxygen species generation as criteria for the diagnosis of infertility.
Am J Obstet Gynecol 1991;164:542-51.
- 37. Griveau JF, Le Lannou D. Reactive oxygen species
and human spermatozoa: physiology and pathology. Int J Androl 1997;20:61-9.
- Sakkas D, Mariethoz E, Manicardi G, Bizzaro D, Bianchi PG, Bianchi U. Origin
of DNA damage in ejaculated human spermatozoa. Rev Reprod 1999;4:31-7.
- Loft S, Kold-Jensen T, HjollundNH, Giwercman A, Gyllemborg J, Ernst E,
et al. Oxidative DNA damage in human sperm influences time to pregnancy.
Hum Reprod
2003;18:1265-72.
- Pasqualotto FF, Sharma RK, Potts JM, Nelson DR, Thomas AJ, Agarwal A. Seminal
oxidative stress in patients with chronic prostatitis. Urology 2000;55:881-5.
- Potts JM, Sharma R, Pasqualotto F, Nelson D, Hall G, Agarwal A. Association
of ureaplasma urealyticum with abnormal reactive oxygen species levels
and absence of leukocytospermia. J Urol 2000;163:1775-8.
- Vicari E. Effectiveness and limits of antimicrobial treatment on seminal
leukocyte concentration and related reactive oxygen species production in
patients with male accessory gland infection. Hum Reprod 2000;15:2536-44.
- de Lamirande E, Leduc BE, Iwasaki A, Hassouna M, Gagnon C. Increased reactive
oxygen species formation in semen of patients with spinal cord injury. Fertil
Steril 1995;63:637-42.
- Padron OF, Brackett NL, Sharma RK, Lynne CM, Thomas AJ, Jr., Agarwal A.
Seminal reactive oxygen species and sperm motility and morphology in men
with spinal
cord injury. Fertil Steril 1997;67:1115-20.
- Naughton CK, NangiaAK, Agarwal A. Pathophysiology of varicoceles in male
infertility. Hum Reprod Update 2001;7:473-81.
- Allamaneni SSR, Naughton CK, Sharma RK, Thomas AJ, Jr., Agarwal A. Increased
seminal reactive oxygen species levels in varicocele patients correlate with
varicocele grade, not testis size. Fertil Steril 2004 (In press).
- Hendin BN, Kolettis PN, Sharma RK, Thomas AJ, Jr., Agarwal A. Varicocele
is associated with elevated spermatozoal reactive oxygen species production
and diminished seminal plasma antioxidant capacity. J Urol 1999;161:1831-4.
- Barbieri ER, Hidalgo ME, Venegas A, Smith R, Lissi EA. Varicocele-associated
decrease in antioxidant defenses. J Androl 1999;20:713-7.
- Mostafa T, Anis TH, El-Nashar A, Imam H, OthmanIA. Varicocelectomy reduces
reactive oxygen species levels and increases antioxidant activity of seminal
plasma from infertile men with varicocele. Int J Androl 2001;24:261-5.
- Kolettis PN, Sharma RK, Pasqualotto FF, Nelson D, Thomas AJ, Jr., Agarwal
A. Effect of seminal oxidative stress on fertility after vasectomy reversal.
Fertil Steril 1999;71:249-55.
- Shapiro RH, Muller CH, Chen G, Berger RE. Vasectomy reversal associated
with increased reactive oxygen species production by seminal fluid leukocytes
and
sperm. J Urol 1998;160:1341-6.
- Saleh RA, Agarwal A, Sharma RK, Nelson DR, Thomas AJ, Jr. Effect of cigarette
smoking on levels of seminal oxidative stress in infertile men: a prospective
study. Fertil Steril 2002;78:491-9.
- Jozwik M, Wolczynski S, Szamatowicz M. Oxidative stress markers in preovulatory
follicular fluid in humans. Mol Hum Reprod 1999;5:409-13.
- Attaran M, Pasqualotto E, Falcone T, Goldberg JM, Miller KF, Agarwal A,
et al. The effect of follicular fluid reactive oxygen species on the outcome
of in vitro fertilization. Int J Fertil Womens Med 2000;45:314-20.
- Paszkowski T, Clarke RN, Hornstein MD. Smoking induces oxidative
stress inside the Graafian follicle. Hum Reprod 2002;17:921-5.
- Guerin P, El Mouatassim S, Menezo Y. Oxidative stress and protection against
reactive oxygen species in the pre-implantation embryo and its surroundings.
Hum Reprod Update 2001;7:175-89.
- Bedaiwy MA, Falcone T, Sharma RK, Goldberg JM, Attaran M, Nelson DR, et
al. Prediction of endometriosis with serum and peritoneal fluid markers:
a prospective controlled trial. Hum Reprod 2002;17:426-31.
- Riley JC, Behrman HR. Oxygen radicals and reactive oxygen species in reproduction.
Proc Soc Exp Biol Med 1991;198:781-91.
- Sugino N, Karube-Harada A, Taketani T, Sakata A, Nakamura Y. Withdrawal
of Ovarian Steroids Stimulates Prostaglandin F2alpha Production Through Nuclear
Factor-kappaB Activation via Oxygen Radicals in Human Endometrial Stromal
Cells:
Potential Relevance to Menstruation. J Reprod Dev 2004;50:215-25.
- Paszkowski T, Traub AI, Robinson SY, McMaster D. Selenium dependent glutathione
peroxidase activity in human follicular fluid. Clin Chim Acta 1995;236:173-80.
- Oyawoye O, Abdel Gadir A, Garner A, Constantinovici N, Perrett C, Hardiman
P. Antioxidants and reactive oxygen species in follicular fluid of women
undergoing IVF: relationship to outcome. Hum Reprod 2003;18:2270-4.
- Wang Y, Sharma RK, Falcone T, Goldberg J, Agarwal A. Importance of reactive
oxygen species in the peritoneal fluid of women with endometriosis or idiopathic
infertility. Fertil Steril 1997;68:826-30.
- Polak G, Koziol-Montewka M, Gogacz M, Blaszkowska I, Kotarski J. Total
antioxidant status of peritoneal fluid in infertile women. Eur J Obstet Gynecol
Reprod Biol 2001;94:261-3.
- Bedaiwy MA, Falcone T. Peritoneal fluid environment in endometriosis.
Clinicopathological implications. Minerva Ginecol 2003;55:333-45.
- Ota H, Igarashi S, Hatazawa J, Tanaka T. Endothelial nitric oxide synthase
in the endometrium during the menstrual cycle in patients with endometriosis
and adenomyosis. Fertil Steril 1998;69:303-8.
- Zeller JM, Henig I, Radwanska E, Dmowski WP. Enhancement of human monocyte
and peritoneal macrophage chemiluminescence activities in women with endometriosis.
Am J Reprod Immunol Microbiol 1987;13:78-82.
- Van Langendonckt A, Casanas-Roux F, Donnez J. Oxidative stress and peritoneal
endometriosis. Fertil Steril 2002;77:861-70.
- Murphy AA, Santanam N, Parthasarathy S. Endometriosis: a disease
of oxidative stress? Semin Reprod Endocrinol 1998;16:263-73.
- Strandell A, Lindhard A. Why does hydrosalpinx reduce fertility? The importance
of hydrosalpinx fluid. Hum Reprod 2002;17:1141-5.
- Bedaiwy MA, Goldberg JM, Falcone T, Singh M, Nelson D, Azab H, et al.
Relationship between oxidative stress and embryotoxicity of hydrosalpingeal
fluid. Hum Reprod 2002;17:601-4.
- Nasr-Esfahani MH, Winston NJ, Johnson MH. Effects of glucose, glutamine,
ethylenediaminetetraacetic acid and oxygen tension on the concentration of
reactive oxygen species and on development of the mouse preimplantation embryo
in vitro. J Reprod Fertil 1992;96:219-31.
- Goto Y, Noda Y, Mori T, Nakano M. Increased generation of reactive oxygen
species in embryos cultured in vitro. Free Radic Biol Med 1993;15:69-75.
- Yang HW, Hwang KJ, Kwon HC, Kim HS, Choi KW, Oh KS. Detection of reactive
oxygen species (ROS) and apoptosis in human fragmented embryos. Hum Reprod
1998;13:998-1002.
- Guyader-Joly C, Guerin P, Renard JP, Guillaud J, Ponchon S, Menezo Y.
Precursors of taurine in female genital tract: effects on developmental capacity
of bovine embryo produced in vitro. Amino Acids 1998;15:27-42.
- Paszkowski T, Clarke RN. The Graafian follicle is a site of L-ascorbate
accumulation. J Assist Reprod Genet 1999;16:41-5.
- Bedaiwy MA, Falcone T, Al-Hussaini T, Abdel-Aleem A, Sharma RK, Worley
SE, et al. Differential growth of human embryos in vitro: role of reactive
oxygen
species. Fertil Steril 2004;82:593-600
- Sukcharoen N, Keith J, Irvine DS, Aitken RJ. Predicting the fertilizing
potential of human sperm suspensions in vitro: importance of sperm morphology
and leukocyte
contamination. Fertil Steril 1995;63:1293-300.
- Zorn B, Vidmar G, Meden-Vrtovec H. Seminal reactive oxygen species as
predictors of fertilization, embryo quality and pregnancy rates after conventional
in vitro fertilization and intracytoplasmic sperm injection. Int J Androl
2003;26:279-85.
- Alvarez JG. Nurture vs nature: how can we optimize sperm quality? J Androl
2003;24:640-8.
- Agarwal A, Nallella KP, Allamaneni SSR, Said TM. Role of antioxidants
in treatment of male infertility: an overview of the literature. Reprod Biomed
Online 2004;8:616-27.
- Suleiman SA, Ali ME, Zaki ZM, el-Malik EM, Nasr MA. Lipid peroxidation
and human sperm motility: protective role of vitamin E. J Androl 1996;17:530-7.
- Vezina D, Mauffette F, Roberts KD, Bleau G. Selenium-vitamin E supplementation
in infertile men. Effects on semen parameters and micronutrient levels
and distribution. Biol Trace Elem Res 1996;53:65-83.
- Lenzi A, Picardo M, Gandini L, Lombardo F, Terminali O, Passi S, et al.
Glutathione treatment of dyspermia: effect on the lipoperoxidation process.
Hum Reprod
1994;9:2044-50.
- Comhaire FH, Christophe AB, Zalata AA, Dhooge WS, Mahmoud AM, Depuydt
CE. The effects of combined conventional treatment, oral antioxidants and
essential fatty acids on sperm biology in subfertile men. Prostaglandins
Leukot Essent Fatty Acids 2000;63:159-65.
- Vicari E, Calogero AE. Effects of treatment with carnitines in infertile
patients with prostato-vesiculo-epididymitis. Hum Reprod 2001;16:2338-42.
- Vicari E, La Vignera S, Calogero AE. Antioxidant treatment with carnitines
is effective in infertile patients with prostatovesiculoepididymitis and
elevated seminal leukocyte concentrations after treatment with nonsteroidal
anti-inflammatory compounds. Fertil Steril 2002;78:1203-8.
- Lenzi A, Lombardo F, Sgro P, Salacone P, Caponecchia L, Dondero F, et
al. Use of carnitine therapy in selected cases of male factor infertility:
a double-blind crossover trial. Fertil Steril 2003;79:292-300.
- Lewin A, Lavon H. The effect of coenzyme Q10 on sperm motility and function.
Mol Aspects Med 1997;18 Suppl:S213-9.
- Henmi H, Endo T, Kitajima Y, Manase K, Hata H, Kudo R. Effects of ascorbic
acid supplementation on serum progesterone levels in patients with a luteal
phase defect. Fertil Steril 2003;80:459-61.
- Crha I, Hruba D, Ventruba P, Fiala J, Totusek J, Visnova H. Ascorbic acid
and infertility treatment. Cent Eur J Public Health 2003;11:63-7.
- Catt JW, Henman M. Toxic effects of oxygen on human embryo development.
Hum Reprod 2000;15 Suppl 2:199-206.
- Wang X, Falcone T, Attaran M, Goldberg JM, Agarwal A, Sharma RK. Vitamin
C and vitamin E supplementation reduce oxidative stress-induced embryo toxicity
and improve the blastocyst development rate. Fertil Steril 2002;78:1272-7.
Copyright © Middle East Fertility Society
|