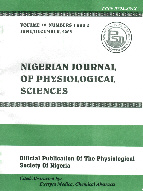
|
Nigerian Journal of Physiological Sciences
Physiological Society of Nigeria
ISSN: 0794-859X
Vol. 20, Num. 1-2, 2005, pp. 19-29
|
Nigerian Journal of Physiological Sciences, Vol. 20, No. 1-2, 2005,
pp. 19-29
CURRENT DEVELOPMENTS IN THE PHYSIOLOGY AND MANAGEMENT
OF ASTHMA
V. I. IYAWE and M. I. EBOMOYI
Department of Physiology, College of Medical Sciences,
University of Benin, Benin City, Nigeria.
Code Number: np05004
Introduction
Asthma is one of the classic diseases recognized by
Hippocrates over 2000 years ago, yet it remains a serious global health problem
because it is still not yet well understood. Asthma prevalence is reported to
be on the increase worldwide especially among children (Kemp, 2003). Fortunately,
recent advances in science have improved our understanding of asthma and the
ability to manage it effectively (GINA, 2004) although deaths from asthma far
from disappearing, have become more frequent in some countries (Jackson et
al, 1982).
Previously, most
information on the pathology of asthma was obtained from postmortem tissue.
Macroscopically, in patients that died of asthma the lungs are over inflated,
with both large and small airways filled with plugs comprised of a mixture of
mucus, serum proteins, inflammatory cells, and cell debris. Microscopically,
the findings are usually of extensive infiltration of the airway lumen and wall
with eosinophils and lymphocytes, vasodilatation, evidence of micro vascular
leakage and epithelial disruption (Dunnil, 1960; Krait et al, 1996).
More recently, studies of living subjects with asthma
have involved endobronchial biopsies of patients with mild disease, which have
generally reflected the findings at autopsy. However studies in patients with
more severe asthma suggest that in addition to eosinophils and lymphocytes,
neutrophils are also present and may play a role in more severe disease (Wenzel
et al, 1997).
Currently, the application of immunological and
molecular biological techniques to asthma has greatly improved understanding of
the disease. This has placed T lymphocytes as pivotal cells in orchestrating
the inflammatory response through the release of multifunctional cytokines
(Robinson et al, 1992). In addition to potent mediators that contract
airway smooth muscle, increase micro vascular leakage, activate different
neurons and stimulate mucus secreting cells, a number of factors are released
that have the capacity to produce structural changes in the airways or attract
inflammatory cells to cause injury to the bronchial tissue. Chemokines have
also been shown to play a crucial role in the recruitment of inflammatory cells
to the airways (Bousquet et al, 2000).
Airway Remodeling
Asthma is currently recognized as a chronic
inflammatory disorder of the airways that leads to tissue injury and subsequent
structural changes collectively called airway remodeling (Homer and Elias,
2005). The inflammatory process in asthma is dominated by so called Th2
inflammation, characterized by T cells that make interleukin (IL) 4, - 5, and
13 along with the classically described eosinophil - , mast cell - , basophil
- , and macrophage rich inflammation (Cohn et al, 2004). This is in
contrast to Th1 T cells, which make interferon - γ ,
lymphotoxin, and IL 2. Th1 and Th2 cells differentiate into polarized
populations from a common precursor on the basis of signals from the local
microenvironment. After they have developed, Th1 and Th2 cells are commonly
thought to inhibit the development of the other cell type. In addition, there
are regulatory cells that also appear to regulate the appearance of each cell
type. Th1 cells play a dominant role in controlling intracellular pathogens
like tuberculosis, whereas Th2 cells play a dominant role in controlling
extracellular pathogens like parasites and mites, and allergens like dusts and
pollens (Alakija et al, 1990). However, the absence of either population
leads to enhanced immunopathology, even in conditions classically thought to
depend on the other cell type.
It is thought that inflammation alone may lead to some
features of asthma, including reversible bronchospasm. However, as with many
chronic inflammatory disorders, asthmatic airway inflammation is also believed
to cause tissue injury and subsequent structural changes. These changes are
referred to collectively as airway remodeling and include 1) an increase
in overall wall thickness, 2) an increase in airway fibrosis and 3) smooth
muscle mass, 4) abnormalities in composition of the extracellular matrix, and
5) an increase in vascularity (Homer and Elias, 2000). These changes have
attracted interest due to the increased realization that these changes may
account for aspects of asthmatic physiology that are poorly addressed with
current anti inflammatory strategies (Busse at al, 2004).
Analysis
and Studies on Airway Remodeling
In fatal asthma, pathological analysis has shown that
most elements of the airway wall (smooth muscle, non smooth muscle connective
tissue, mucus glands) are increased (Carroll et al, 1993; Huber and
Koessler, 1922; James et al, 1989; Kuwano et al, 1993). The
changes (except for the increase in mucus glands) are found in airways of all
sizes (Saetta et al, 1991). The pathological changes in airways of
patients with nonfatal asthma are much less pronounced, with changes seen
predominantly in small airways (2 4 mm in diameter) (Carroll et al,
1997; Kuwano et al, 1993). Airway wall thickness as measured
radiologically also correlates with disease severity and length of time with
disease, with small airways again being the predominant abnormality in milder
disease (Awadh et al, 1998; Boulet et al, 1995; Goldin et al,
1998; Harmanci et al, 2002; Kasahara et al, 2002; Little et
al, 2002; Okazawa et al, 1996 and Paganin et al, 1996).
An increase in airway smooth muscle mass is one of the
best known features of asthmatic remodeling (Huber and Koessler, 1922). Like
total wall thickness, smooth muscle thickness correlates with fatal or nonfatal
asthma (Carroll et al, 1993; James et al, 1989 and Kuwano et
al, 1993). The degree to which hyperplasia compared with hypertrophy
contributes to this response continues to be controversial, but both probably
contribute in different patient populations (Benayoun et al, 2003; Ebina
et al, 1993, and Woodruff et al, 2004). The degree to which this
increased smooth muscle is abnormal is also controversial (Benayoun et al,
2003 and Woodruff et al, 2004).
An increase in fibrosis just
below the large airway epithelium occurs as a prominent feature in asthma. This
layer has been extensively studied due to its accessibility on endobronchial
biopsy. The normal layer of collagen under the airway is ~ 5 μm
thick, which increases to 7 23 μm in
patients with asthma. Initially described as basement membrane thickening, it is
now apparent that the true basement membrane (the lamina rara and densa as seen
on electron microscopy, which contain laminin and collagen IV) is not grossly
altered (Roche et al, 1989). There is, rather, thickening of the area
just below the true basement membrane, the lamina reticularis, with deposition
of interstitial collagens I, III, and V. Studies have reported correlations of
subepithelial fibrosis as measured in larger cartilaginous airways with overall
wall thickness in the same airways but not in small airways elsewhere in the
same lung (James et al, 2002 and Kasahara et al, 2002). There are
inconsistent results with respect to enhanced collagen deposition in the
submucosa (area between the layer of dense subepithelial fibrosis and smooth
muscle) (Benayoun et al, 2003; Chu et al, 1998 and Wilson and Li,
1997). Abnormalities have also been noted in noncollagenous matrix, including
elastin, proteoglycans, and cartilage (Lazaar and Panettieri, 2003).
The significance of this specific feature of asthmatic
airway remodeling is unclear. Although measurements of airway distensibility
correlate well with subepithelial fibrosis (Ward et al, 2001), other
functional measurements (clinical illness scores, measures of pulmonary
function and airway hyperresponsiveness) show variable correlations (Benayoun et
al, 2003; Boulet et al, 1997; Chetta et al, 1997; Cho et
al, 1996 and Hoshina et al, 1998). Other groups have identified
severe asthmatics with no increase in subepithelial fibrosis (Chakir et al,
2003; Chu et al, 1998 and Wenzel et al, 1999). Subepithelial
fibrosis is actually a very early marker for the asthmatic phenotype in
children and does not correlate with length of time with the disease nor
necessarily with the severity of inflammation (Boulet et al, 2000; Chu et
al, 1998; Cokugras et al, 2001; Laitinen et al, 1996 and
Payne et al, 2003). It has therefore been suggested that subepithelial
fibrosis represents disordered epithelial mesenchymal signaling rather than a
direct response to inflammatory injury (Holgate et al, 2004). A tracheal
explant model has shown that cigarette smoke can induce remodeling in the
absence of inflammation, indicating that other pathways to fibrosis need to be
considered (Wang et al, 2003 and Iyawe et al, 2005).
Myofibroblasts are specialized
cells with features of both fibroblasts and myocytes. They have the synthetic
machinery of fibroblasts used for synthesis of extracellular matrix but also
have at least some components of the contractile apparatus of myocytes. They
are well known to be increased in tissues undergoing repair, such as in wounds
or in pulmonary interstitial fibrosis. In human asthma, the submucosa shows an
increase in myofibroblasts that correlates with the thickness of the lamina
reticularis but not with severity of disease (Benayoun et al, 2003;
Brewster et al, 1990 and Hoshino et al, 1998). Since
myofibroblasts are well known sources of interstitial collagens, it is
plausible to suggest that the myofibroblasts are the source of the
subepithelial fibrosis. The origin and fate of these cells is somewhat obscure.
Cells with this phenotype appear very quickly after antigen challenge, possibly
implying a quiescent precursor cell that acquires myofibroblastic markers
without necessarily dividing (Gizycki et al, 1997). Other data suggest
that these cells may arise from circulating precursors (Schmidt et al,
2003).
Increased vascularity
is a common feature of chronic inflammation. In addition, increased vascular
congestion, leading to wall thickening, has been suggested as the basis of exercise
induced asthma, the airway hyperresponsiveness seen in congestive heart failure
and in normal
subjects after a rapid infusion of intravenous fluids (Cabanes et al, 1989
and Rolla et al, 1986). More severe asthmatics have a greater number of
vessels than milder asthmatics (Salvato, 2001 and Vrugt et al, 2000).
Increased vascularity is noted in both newly diagnosed patients and in patients
with long standing asthma. There is a correlation of vascularity with airway
hyperresponsiveness and change in lung function after bronchodilator treatment
(Hoshino et al, 2001 and Orsida et al, 1999). The vessels that
are increased appear to be capillaries and venules and are concentrated just
under the airway epithelium (Orsida et al, 2001 and Salvato, 2001). Abnormalities
of various types of vessels in the airway mucosa have also been described. (Salvato,
2001). Paradoxically, but consistent with results measuring other aspects of
airway remodeling, there is no correlation of degree of abnormality with length
of time with disease. As in animal models, in patients, vascularity is denser
in intercartilagenous areas
than over cartilage (McDonald, 2001).
Mechanisms of Airway Remodeling
A large number of mediators have been described in
airways of asthmatics that could theoretically be relevant to airway
remodeling. In many cases, the presence of these mediators correlates with the
severity of airway remodeling or some clinical feature. However, it is
difficult to know the significance of these findings in the absence of a
specific inhibitor of that mediator. So far, only one specific mediator, IL5,
has been targeted in humans. IL-5 was well established as potentially relevant
to asthma on account of its powerful influence on eosinophil development and
priming. Treatment of asthmatics with a humanized anti IL-5 antibody
confirmed this suspicion, because it caused a marked reduction in circulating
and airway lumen eosinophils and a lesser reduction in airway tissue
eosinophils. Furthermore, treatment with this antibody was able to reduce
matrix proteins present beneath the epithelial basement such as tenascin C and
lumican (Flood-Page et al, 2003). Whether this effect of anti IL-5 on
matrix turnover is eosinophil dependent is not known because airway fibroblasts
and epithelial cells also possess IL-5 receptors. Unfortunately, despite these
impressive biological effects, the antibody failed to produce any clinically
significant effect on asthmatic physiology.
IL-13 has been shown to be critically important in
acute models of allergic inflammation (Wills-Karp et al, 1998). It was
originally discovered as an IL-4 like molecule with which it shares some
receptor subunits. It has since become clear that IL-13 is more important in
the effector phase and that IL-4 is more important in the initiation phase of
Th2 inflammation. With the use of both conventional and inducible transgenic
modeling, IL-13 was shown to be a potent inducer of an eosinophils -,
macrophage -, and lymphocyte rich inflammatory response, airway fibrosis,
mucus metaplasia, and airway hyperresponsiveness (Zhu et al, 1999). IL-13
mediates many of its effects through a signal transducer and activator of
transcription (STAT)-6 signaling pathway, and human asthmatics have elevated
levels of STAT-6 in airway epithelium (Mullings et al, 2000).
Collagen deposition in tissues is controlled by the
balance of the collagen degrading matrix metalloproteinases (MMPs) and their
inhibitors, the tissue inhibitors of metalloproteinases (TIMPs). In addition to
their role in matrix remodeling, it has also become apparent that MMPs have
important roles in inflammation, angiogenesis, and cell cell signaling (Kelly
and Jarjour, 2003). In asthma, the potentially most important members of this family
are MMP-9 and TIMP-1. MMP-9 is produced in an exaggerated fashion by asthmatic
alveolar macrophages and is found in exaggerated quantities in sputum,
bronchoalveolar lavage (BAL) fluid, and biopsies from patients with asthma. The
ratio of MMP-9 and TIMP-1 in asthmatics is lower than in control subjects and
correlates with the degree of airway obstruction (Kelly and Jarjour, 2003).
Other Th2 cytokines, such as IL-9 and IL-10, appear to
function at least partly via inducing IL-13 (Lee et al, 2002 and
Whittaker et al, 2002). IL-10 has been considered in some cases a Th2
cytokine but has also been implicated in immunoregulation. Mice that
overexpress IL-10 in the airway show a reduction in aspects of innate immunity,
including endotoxin induced tumor necrosis factor production and neutrophil
accumulation. However, IL-10 also causes mucus metaplasia, B cell - and T cell
rich inflammation, and airway fibrosis and augments the levels of mRNA
encoding Gob-5, mucins, and IL-13. These responses are mediated by multiple
mechanisms, with mucus metaplasia being dependent on an IL-13-IL-4Ra-STAT-6 pathway, whereas the inflammation and fibrosis were independent
of that pathway (Lee et al, 2002).
The most heavily studied mediator of tissue fibrosis
is transforming growth factor (TGF)-β1. TGF-β1 is made by many cells within the lungs, such as epithelial cells,
macrophages, eosinophils, lymphocytes, and fibroblasts. TGF-β1 can induce fibroblast and smooth muscle proliferation and enhances
matrix production. TGF-β1 mRNA appears to be increased in moderate and severe
asthmatics compared with normal subjects, and the expression of this cytokine
is directly related to the degree of subepithelial fibrosis (Minshall et al,
2000 and Ohno et al, 1996). As determined by using in situ
hybridization, eosinophils and fibroblasts are the principal cells synthesizing
TGF-β1 in the airway, whereas alveolar macrophages release
more TGF-β 1 from asthmatics than from controls (Hoshino et
al, 1998; Vignola et al, 1997 and Vignola et al, 1996).
Expression of downstream signaling molecules from TGF-β also shows an increase in asthma and a correlation with subepithelial
fibrosis (Nakao et al, 2000 and Sagara et al, 2002). Macrophages
were the major site of TGFb-1 production as assessed by
immunohistochemistry and in situ hybridization. IL-13-induced fibrosis was
significantly ameliorated by treatment with the TGF-β antagonist soluble TGFβR-Fc (Lee et al, 2001)..
It is possible, therefore, that the main significance of MMP-9 overexpression in
human asthma may be to activate TGF-β1 (Lee et al,
2001).
A large number of factors are
known that control airway smooth muscle cell proliferation in vitro
(Panettieri, 2003). However, little is certain about their role in vivo. IL-11,
a member of the IL-6 type cytokine family, shares a common receptor
signaling subunit with IL-6 and has been shown to induce airway
hyperresponsiveness in mice when administered intratracheally (Einarsson et
al, 1996 ). It is produced by a variety of lung stromal cells, including
epithelial cells, smooth muscle cells, and fibroblasts, in response to a
variety of stimuli, including asthma related viruses, histamine, and
eosinophils major basic protein (Einarsson et al, 1996 and Elias et
al, 1994). Overexpression of IL-11 in the lungs caused an increase in
peribronchial fibrosis and an increase in true airway smooth muscle mass and
myofibroblasts (Tang et al, 1996). There is an increase in baseline
airway resistance and marked airway hyperresponsiveness. The studies with the
IL-11 mice led to analysis of human airways that showed that IL-11 is
overexpressed in chronic human asthma but only in the most severely remodeled
airways (Minshall et al, 1999).
Increased vascularity may be due to any number of
proangiogenic factors, especially vascular endothelial growth factor (VEGF).
VEGF was originally described as vascular permeability factor on the basis of
its ability to generate tissue edema. It has subsequently been appreciated to
be a multi functional angiogenic regulator that stimulates epithelial cell
proliferation, blood vessel formation, and endothelial cell survival (Clauss,
2000 and Gerber et al, 1998). VEGF levels are increased in asthmatics,
and levels correlate directly with disease activity and inversely with airway
caliber and airway responsiveness (Asai et al, 2002; Hoshino et al,
2001 and Lee and Lee, 2001). VEGF has been postulated to contribute to
asthmatic tissue edema via its vascular permeability factor effect (Anthony et
al, 2002 and Thurston et al, 2000). IL-13 is known to cause an
increase in VEGF production (Corne et al, 2000). In mice that over -
express VEGF, there is a dramatic endothelial sprouting under the airway
epithelium (Lee et al, 2004), potent induction of angiogenesis and edema
in the airway and lung. There is evidence for remodeling, with an increase in
smooth muscle mass around airways. Later there is an increase in collagen
around airways. TGF-β1 was also increased at this latter time point. These
airway and lung changes were accompanied by an increase in airway
hyperresponsiveness.
VEGF also caused a marked increase in inflammation,
with an increase in mononuclear cells, T and B cells, and eosinophils. There
was an increase in the number and activation state of dendritic cells that
correlated with ability to prime the mice via the airways, a feature not found
in wild type mice.
As expected from the IL-13
over expressing mice, after allergen challenge, VEGF was found to be markedly
increased in BAL fluids. In the lung, VEGF is localized to airway epithelial
cells, mononuclear cells, and T cells. In vitro, polarized Th2 cells are much
more potent producers of VEGF than Th1 cells. When a VEGF receptor antagonist
was used in vivo, there was a dramatic decrease in BAL and tissue inflammation
as well as airway hyper responsiveness. There was also a decrease in IL-13 and
IL-4
production (Lee et al, 2004).
These results suggest a positive feed forward
circuit in which allergen challenge increases VEGF while VEGF also increases
allergic inflammation, dendritic cell activation and airway remodeling. It is
thought that viral infection early in life can predispose one to the
development of asthma (Schwarze and Gelfand, 2002 and Sigurs et al,
2000). Because viral infection can also lead to increased VEGF (Einarsson et
al, 1996), these results suggest a mechanism whereby this may occur. Finally,
given the ability of VEGF to promote both inflammation and airway remodeling,
this data supports a possible role for VEGF antagonists in therapy of asthma, especially
in severe asthma, which is resistant to conventional therapy.
Physiological Significance of Airway Remodeling
Airway remodeling has been invoked to explain various
aspects of asthma severity, including airway hyper responsiveness and fixed airway
obstruction (Busse et al, 2004). The cross sectional studies comparing
fatal and nonfatal asthma certainly support the general concept of a
relationship between airway remodeling and asthma severity. Although
experimental studies of allergic models show that airway hyper responsiveness
can be induced without airway remodeling (Hoshino et al, 1998) and
individual cytokines are known that can increase airway smooth muscle
responsiveness both in vitro and in vivo (Amrani and Panettieri, 1998 and
Einarsson et al, 1996) a murine model of airway remodeling suggests that
airway dysfunction persists after resolution of inflammation, implicating the
airway remodeling itself in airway dysfunction (Leigh et al, 2002).
Extensive mathematical modeling also suggests effects of airway remodeling on
airway function similar to those seen clinically (Okazawa et al, 1996).
Fixed airway obstruction associated with progressive
loss of lung function has now been demonstrated in several cohort studies of
both adults and children (Covar et al, 2004; Lang et al, 1998;
Peat et al, 1987 and Ulrik et al, 1992). Although the
pathological basis for this phenomenon is not completely known, studies have
shown a remarkable correlation of asthma severity with measures of smooth
muscle area, smooth muscle hypertrophy, and density of fibroblasts in the
airway wall (Benayoun et al, 2003). Interestingly, another group had not
only not seen this effect, they also saw a correlation of subepithelial
fibrosis with disease severity, which was specifically not seen by the first
group (Cho et al, 1996). It is possible that this represents disease
heterogeneity.
A number of papers have addressed the issue of
reversibility of airway remodeling. Early studies showed no effect of steroids
on subepithelial fibrosis (Jeffrey et al, 1992; Lundgren et al,
1988 and Roche et al, 1989). More recent randomized studies have shown a
significant reduction in subepithelial fibrosis after short term or long
term therapy or after withdrawal from exposure to antigen (Chetta et al,
1997; Hoshino et al, 1998; Saetta et al, 1995 and Ward et al,
2002). It has been suggested that prior treatment with steroids, delayed
treatment, or low doses and short courses of steroids prevented an effect from
being noted in the earlier studies. Steroids also decrease the number of
(myo)fibroblasts in the submucosa and reduce airway vascularity (Chetta et
al, 2003; Hoshino et al, 1998; Hoshino et al, 2001 and Orsida
et al, 1999). The decrease in vessel number correlates with the decrease
in airway hyper responsiveness, reduction in inflammation, and change in
subepithelial collagen (Chetta et al, 2003 and Hoshino et al,
2001). One study suggests that addition of a β2 agonist
to inhaled steroids may reduce vascularity further (Orsida et al, 2001).
These pathological studies have been used to support the suggestion from
clinical trials that early treatment with inhaled steroids is required to
prevent irreversible loss of lung function, at least in adults (Haahtela et
al, 1994; Overbeek et al, 1996 and Selroos et al, 1995).
Recent advances in the treatment and management of
asthma have shown that leukotriene (LT) receptor antagonists or LT modifiers
are very beneficial as a second generation therapy with steroid sparing
properties and negligible side effects (Moqbel, 1999, and Mission et al,
1999).
A few studies have addressed the issue of response of
putative remodeling mediators to therapy. TGF-β appears
resistant to steroid therapy, whether there is a reduction in measures of
airway remodeling or not (Chakir et al, 2003 and Hoshino et al,
1998). However, since remodeling presumably requires more than one mediator and
because alternative pathways are likely, it is hard to interpret this kind of
data.
There remain many areas of
uncertainty in this field. Despite the suggestion that airway remodeling
explains the lack of response to therapy of some patients, no study has
specifically shown that those patients who either fail to respond to therapy or
progress despite therapy do in fact show airway remodeling (or excess
expression of some mediator of remodeling) that fails to respond or progresses
despite a reduction in inflammation. No prospective study has examined the
effects of therapy on smooth muscle. Finally, one explanation for the disparate
results discussed here is that of clinical heterogeneity, i.e., different
subpopulations of patients have different aspects of airway remodeling related
to their particular physiology. If this hypothesis was true, it would
ultimately be necessary to characterize the pathological basis of each
patients physiology before determining which therapy would be most beneficial
in reversing or preventing airway remodeling in that individual patient. This
field remains open for research, especially in relation to treatment.
References
-
Alakija, W; Iyawe, V.I; Jarikre, L.N.
and Chiwuzie, J.C. (1990). Ventilatory function of workers at Okpella Cement
Factory. W. Afr. J. Med. 9, 187 192.
-
Amrani, Y, and Panettieri, R.J. (1998).
Cytokines induce airway smooth muscle cell hyperresponsiveness to contractile
agonists. Thorax. 53, 713 716.
-
Anthony, A.B; Tepper, R.S, and Mohammed,
K.A. (2002). Cockroach extract antigen increases bronchial airway epithelial
permeability. J. Allergy. Clin. Immunol. 110, 589 595.
-
Asia, K; Kanazawa, H; Otani, K;
Shiraishi, S; Hirata, K, and Yoshikawa, J. (2002). Imbalance between vascular
endothelial growth factor and endostatin levels in induced sputum from
asthmatic subjects. J. Aller. Clin. Immunol. 110, 571 575.
-
Awadh, N., Muller, N., Park, C., Abboud,
R. and FitzGerald, J. (1998). Airway wall thickness in patients with near fatal
asthma and control groups: assessment with high resolution computed tomographic
scanning. Thorax. 53, 248 253.
-
Benayoun, L; Druilhe, A; Dombret, M.C;
Aubier, M. and Pretolani, M. (2003). Airway structural alterations selectively
associated with severe asthma. Am. J. Respir. Crit. Care. Med. 167, 1360
1368.
-
Boulet, L.P., Belanger, M. and Carrier,
G. (1995). Airway responsiveness and bronchial wall thickening in asthma
with or without fixed airflow obstruction. Am. J. Respir. Crit. Care. Med. 152,
865 871.
-
Boulet, L.P; Turcotte, H; Laviolette, M;
Naud, F; Bernier, M.C; Martel, S. and Chakir, J. (2000). Airway
hyperresponsiveness, inflammation, and subepithelial collagen deposition in
recently diagnosed versus long standing mild asthma. Influence of inhaled
corticosteroids. Am. J. Respir. Crit. Care. Med. 162, 1308 1313.
-
Boulet, L; Laviolette, M; Turcotte, H;
Cartier, A; Dugas, M; Malo, J. and Boutet, M. (1997). Bronchial subepithelial
fibrosis correlates with airway responsiveness to methacholine. Chest. 112,
45 52.
-
Bousquet, J., Jeffery, P.K., Busse,
W.W., Johnson, M. and Vignola, A.M. (2000). Asthma: from bronchoconstriction to
airway inflammation and remodeling. Am. J. Respir. Crit. Care. Med. 161,
1720 1745.
-
Busse, W., Banks-Schlegel, S., Noel, P.,
Ortega, H., Taggart, V. and Elias, J. (2004). Future research directions in
asthma: an NHLBI Working Group report. Am. J. Respir. Crit. Care. Med. 170,
683 690.
-
Busse, W; Banks-Schlegel, S; Noel, P;
Ortega, H; Taggart, V, and Elias, J. (2004). Future research directions in
asthma: an NHLBI Working Group report. Am. J. Respir. Crit. Care. Med. 170,
683 690.
-
Cabanes, L; Weber, S; Matran, R; Regnard,
J; Richard, M; DeGeorges, M, and Lockhart, A. (1989). Bronchial
hyperresponsiveness to methacholine in patients with impaired left ventricular
function. N. Eng. J. Med. 106, 721 728.
-
Carroll, N., Elliot, J., Morton, A. and
James, A. (1993). The structure of large and small airways in nonfatal and
fatal asthma. Am. Rev. Respir. Dis. 147, 405 410.
-
Chakir, J; Laviolette, M; Boutet, M;
Laliberte, R; Dube, J. and Boulet, L. (1996). Lower airways remodeling in
nonasthmatic subjects with allergic rhinitis. Lab. Invest. 75, 735
744.
-
Chakir, J; Shannon, J; Molet, S;
Fukakusa, M; Elias, J; Laviolette, M. et al.. (2003). Airway remodeling
associated mediators in moderate to severe asthma: effect of steroids on TGF-b, IL-11, IL-17, and type I and type III collagen expression. J.
Allergy. Clin. Immunol. 111, 1293 1298.
-
Chetta, A; Foresi,
A, Del Donno, M; Bertorelli, G; Pesci, A. and Oliveri, D. (1997). Airways
remodeling is a distinctive feature
of asthma and is related to severity of disease. Chest. 111, 852 857.
-
Chetta, A; Zanini, A; Foresi, A; Del
Donno, M; Castagnaro, A; DIppolito, R. (2003). Vascular component of airway
remodeling in asthma is reduced by high dose of fluticasone. Am. J. Respir.
Crit. Care. Med. 167, 751 757.
-
Cho, S; Seo, J; Choi, D; Yoon, H; Cho,
Y; Min, K. (1996). Pathological changes according to the severity of asthma. Clin.
Exp. Allergy. 26, 1210 1219.
-
Cho, S; Seo, J; Choi, D; Yoon, H; Cho,
Y; Min, K. (1996). Pathological changes according to the severity of asthma. Clin.
Exp. Allergy. 26, 1210 1219.
-
Chu, H; Halliday, J; Martin, R; Leung,
D; Szefler, S. and Wenzel, S. (1998). Collagen deposition in large airways may
not differentiate severe asthma from milder forms of the disease. Am. J.
Respir. Crit. Care. Med. 158, 1936 1944.
-
Clauss, M. (2000). Molecular biology of
the VEGF and the VEGF receptor family. Semin. Thromb. Hemost. 26, 561
569.
-
Cohn, L., Elias, J.A. and Chupp, G.L.
(2004). Asthma: mechanisms of disease persistence and progression. Annu.
Rev. Immunol. 22, 789 815.
-
Cohn, L; Tepper, J.S, and Bottomly, K.
(1998). IL-4 independent induction of airway hyperresponsiveness by Th2, but
not Th1, cells. J. Immunol. 161, 3813 3816.
-
Cokugras, H; Akcakaya, N; Seckin
Camcioglu, Y; Sarimurat, N. and Aksoy, F. (2001). Ultrastructural examination
of bronchial biopsy specimens from children with moderate asthma. Thorax. 56,
25 29.
-
Corne, J; Chupp, G; Lee, C.G; Homer,
R.J; Zhu, Z; Chen, Q. (2000). IL-13 stimulates vascular endothelial growth
factor and protects against hyperoxic acute lung injury. J. Clin. Invest. 106,
783 791.
-
Covar, R.A.; Spahn, J.D; Murphy, J.R,
and Szefler, S.J. (2004). Progression of asthma measured by lung function in
the childhood asthma management program. Am. J. Respir. Care. Med. 170,
234 241.
-
Dunnil, M.S. (1960). The pathology of
asthma with special reference to changes in the bronchial mucosa. J. Clin.
Pathol. 13, 27 33.
-
Ebina, M; Takahashi, T; Chiba, T. and
Motomiya, M. (1993). Cellular hypertrophy and hyperplasia of airway smooth
muscles underlying bronchial asthma. Am. Rev. Respir. Dis. 148, 720
726.
-
Einarsson, O; Geba, G.P; Zhu, Z;
Landry, M, and Elias, J.A. (1996). Interleukin-11: Stimulation in vivo
and in vitro by respiratory viruses and induction of airways hyperresponsiveness. J.
Clin. Invest. 97, 915 924.
-
Elias, J.A; Zheng, T; Einarsson, O;
Landry, M; Trow, T.K; Rebert, N, and Panuska, J. (1994). Epithelial
interleukin-11: regulation by cytokines, respiratory syncytial virus and
retinoic acid. J. Biol. Chem. 169, 22261 22268.
-
Flood-Page, P; Menzies-Gow, A; Phipps,
S; Ying, S; Wangoo, A; Ludwig, M.S. et al. (2003). Anti-IL-5 treatment
reduces deposition of ECM proteins in the bronchial subepithelial basement
membrane of
mild atopic asthmatics. J. Clin. Invest. 112, 1029 1036.
-
Gerber, H.P; Dixit, V, and Ferrara, N.
(1998). Vascular endothelial growth factor induces expression of the
antiapoptotic proteins Bcl-2 and A1 in vascular endothelial cells. J. Biol.
Chem. 273, 13313 13316.
-
Gizycki, M; Adelroth, E; Rogers, A;
OByrne, P. and Jeffery, P. (1997). Myofibroblast involvement in the allergen
induced late response in mild atopic asthma. Am. J. Respir. Cell. Mol. Biol.
16, 664 673.
-
Global Initiative for Asthma. (2004).
Global Strategy for asthma management and prevention. 1 7.
-
Goldin, J; McNitt-Gray, M; Sorenson, S;
Johnson, T; Dauphinee, B; Kleerup, E. (1998). Airway hyperreactivity:
assessment with helical thin section CT. Radiology. 208, 321 329.
-
Haahtela, T; Jarvinen, M; Kava, T;
Kivranta, K; Koskinen, S, and Lehtonen, K. (1994). Effects of reducing or
discontinuing inhaled budesonide in patients with mild asthma. N. Engl. J.
Med. 331, 700 705.
-
Harmanci, E; Kebapci, M; Metintas, M.
and Ozkan, R. (2002). High resolution computed tomography findings are correlated
with disease severity in asthma. Respiration. 69, 420 426.
-
Holgate, S; Holloway, J; Wilson, S;
Bucchieri, F; Puddicombe, S. and Davies, D. (2004). Epithelial mesenchymal
communication in the pathogenesis of chronic asthma. Proc. Am. Thorac. Soc. 1,
93 98.
-
-
Homer, R.J,
and Elias, J.A. (2000). Consequences of
long term inflammation. Airway remodeling. Clin. Chest. Med. 21,
331
343.
-
Homer, R.J. and Elias, J.A. (2000).
Consequences of long term inflammation. Airway remodeling. Clin. Chest.
Med. 21, 331 343.
-
Homer, R.J. and Elias, J.A. (2005).
Airway remodeling in asthma: Therapeutic implications of mechanism. Physiol.
20, 28 - 35.
-
Hoshino, M; Nakamura, Y, and Hamid, Q.A.
(2001). Gene expression of vascular endothelial growth factor and its receptors
and angiogenesis in bronchial asthma. J. Allergy. Clin. Immunol. 107,
1034 1038.
-
Hoshino, M; Nakamura, Y, and Sim, J.
(1998). Expression of growth factors and remodeling of the airway wall in
bronchial asthma. Thorax. 53, 21 27.
-
Hoshino, M; Nakamura, Y; Sim, J;
Shimojo, J. and Isogai, S. (1998). Bronchial subepithelial fibrosis and
expression of matrix metalloproteinase -9 in asthmatic airway inflammation. J.
Allergy. Clin. Immunol. 102, 783 788.
-
Hoshino, M; Nakamura, Y; Sim, J;
Yamashiro, Y; Uchida, K; Hosaka, K, and Isogai, S. (1998). Inhaled
corticosteroid reduced lamina reticularis of the basement membrane by
modulation of insulin growth factor (IGF-1) in bronchial asthma. Clin. Exp.
Allergy. 28, 568 577.
-
Hoshino, M; Takahashi, M, and Aoike, N.
(2001). Expression of vascular endothelial growth factor, basic fibroblast
growth factor, and angiogenin immunoreactivity in asthmatic airways and its
relationship to angiogenesis. J. Allergy. Clin. Immunol. 107, 295 301.
-
Hoshino, M; Takahashi, M; Takai, Y; Sim,
J, and Aoike, N. (2001). Inhaled corticosteroids decrease vascularity of the
bronchial mucosa in patients with asthma. Clin. Exp. Allergy. 31, 722
730.
-
Huber, H.L. and Koessler, K.K. (1922).
The pathology of bronchial asthma. Arch. Intern. Med. 30, 689 760.
-
Iyawe, V.I; Ejindu, C. and Ebomoyi, M.I.
(2005). The effect of cigarette puff on airway responsiveness. Nig. J. Clin.
Biomed. Res. (in press).
-
Jackson, R.T., Beaglehole, R., Rea, H.H.
and Sutherland, D.C. (1982). Asthma mortality: A new epidemic in New Zealand. Br.
Med. J. 285, 771.
-
James, A.L., Pare, P.D. and Hogg, J.C.
(1989). The mechanics of airway narrowing in asthma. Am. Rev. Respir. Dis. 139,
242 246.
-
James, A.L; Maxwell, P.S; Pearce-Pinto,
G; Elliot, J.G. and Carroll, N.G. (2002). The relationship of reticular
basement membrane thickness to airway wall remodeling in asthma. Am. J.
Respir. Crit. Care. Med. 166, 1590 1595.
-
Jeffery, P; Godfrey, R; Adelroth, E;
Nelson, F; Rogers, A, and Johansson, S. (1992). Effects of treatment of airway
inflammation and thickening of basement membrane reticular collagen in asthma. Am.
Rev. Respir. Dis. 145, 890 899.
-
Kasahara, K; Shiba, K; Ozawa, T; Okuda,
K. and Adachi, M. (2002). Correlation between the bronchial subepithelial layer
and whole airway wall thickness in patients with asthma. Thorax. 57, 242
246.
-
Kelly, E.A, and Jarjour, N.N. (2003).
Role of matrix metalloproteinases in asthma. Curr. Opin. Pulm. Med. 9,
28 33.
-
Kemp, J.P. (2003). Recent advances in
the management of Asthma using leukotriene modifiers. Am. J. Respir .Med. 2(2),
139 156.
-
Krait, M., Djukanovic, R., Wilson, S.,
Holgate, S.T. and Martin, R.J. (1996). Alveolar tissue inflammation in asthma. Am.
J. Respir. Crit. Care. Med. 154, 1505 1510.
-
Kuwano, K., Bosken, C., Pare, P., Bai,
R., Wiggs, B. and Hogg, J. (1993). Small airways dimensions in asthma and in
chronic obstructive pulmonary disease. Am. Rev. Respir. Dis. 148, 1220
1225.
-
Laitinen, L.A; Laitinen, A; Altraja, A;
Virtanen, I; Kampe, M; Simonsson, B.G. (1996). Bronchial biopsy findings in
intermittent or early asthma. J. Allergy. Clin. Immunol. 98, S3 S6,
discussion S33 S40.
-
Lange, P; Parner, J; Vestbo, J; Schnohr,
P, and Jensen, G.A. (1998). A 15-year follow up study of ventilatory function
in adults with asthma. N. Engli. J. .Med. 339, 1194 1200.
-
Lazaar, A.L. and Panettieri, R.A. Jr.
(2003). Is airway remodeling clinically relevant in asthma? Am. J. Med. 115,
652 659.
-
Lee, C.G; Homer, R.J; Zhu, Z; Lanone, S;
Wang, X; Koteliansky, V..(2001). Interleukin (IL)- 13 induces tissue fibrosis
by selectively stimulating and activating transforming growth factor b(1). J. Exp. Med. 194, 809 822.
-
Lee, C.G; Link, H; Baluk, P; Homer, R.
J; Chapoval, S; Bhandari, V. (2004). Vascular endothelial growth factor (VEGF)
induces parenchymal and vascular remodeling and plays a critical role in Th2
sensitization and inflammation in the lung. Nat. Med. 10, 1095 1103.
-
-
Lee, Y.C, and
Lee, H.K. (2001). Vascular endothelial
growth factor in patients with acute asthma. J. Allergy. Clin. Immunol. 107,
1106.
-
Leigh, R; Ellis, R; Wattie, J; Southham,
D.S; DeHoogh, M; Gauldie, J. (2002). Dysfunction and remodeling of the mouse
airway persist after resolution of acute allergen induced airway
inflammation. Am. J. Respir. Cell. Mol. Biol. 27, 526 535.
-
Little, S.A; Sproule, M.W; Cowan, M.D;
Macleod, K.J; Robertson, M; Love, J.G. et al. (2002). High resolution computed
tomographic assessment of airway wall thickness in chronic asthma:
reproducibility and relashionship with lung function and severity. Thorax. 57,
247 253.
-
Lundgren, R; Soderberg, M; Horstedt, P.
and Stenling, R. (1988). Morphological studies on bronchial mucosal biopsies
from asthmatics before and after ten years treatment with inhaled steroids. Eur.
Respir. J. 1, 883 889.
-
McDonald, D.M, (2001). Angiogenesis and
remodeling of airway vasculature in chronic inflammation. Am. J. Respir.
Crit. Care. Med. 164, S39 S45.
-
Minshall, E.M.; Chakir, J; Laviolette,
R; Zhu, Z; Elias, J.A, and Hamid, Q.A. (1999). Interleukin-11 mRNA expression
is increased in severe asthmatic localization to eosinophils (Abstract). J.
Allergy. Clin. Immunol. 103, S58.
-
Minshall, E.M; Leung, D.Y; Martin, R.J;
Cameron, L; Ernst, P, and Hamid, Q. (1997). Eosinophil associated TGF-b1 mRNA expression and airway fibrosis in asthma. Am. J. Respir.
Cell. Biol. 17, 326 333.
-
Mission, J; Clark, W. and Kendall, M.J.
(1999).Therapeutic advances: Leukotriene antagonists for the treatment of
asthma. J. Clin. Pharm. Ther. 24(1): 17 22.
-
Moqbel, R. (1999). Leukotriene receptor
antagonists in the treatment of asthma: implications for eosinophil
inflammation. Can. Respirat .J. 6(5), 453 457.
-
Mullings, R.E; Wilson, S.J; Puddicombe,
S.M; Bucchieri, F; Djukanovic, R; Howarth, P.H. et al. (2000). Signal transduction
and activator of transcription 6 (STAT-6) expression and function in asthmatic
bronchial epithelium. J. Allergy. Clin. Immunol. 108, 832 838.
-
Nakao, A; Sagara, H; Setoguchi, Y;
Okumura, K; Ogawa, H, and Fukuda, T. (2000). Expression of Smad7 in bronchial
epithelial cells is inversely correlated to basement membrane thickness and
airway hyperresponsiveness in patients with asthma. J. Allergy. Clin.
Immunol. 110, 873 878.
-
Ohno, I; Nitta, Y; Yamauchi, K; Hoshi,
H; Honma, M; Woolley, K. (1996). Transforming growth factor b1 (TGF b1) gene expression by eosinophils in asthmatic airway
inflammation. Am .J. Respir. Cell. Mol. Biol. 15, 404 409.
-
Okazawa, M; Muller, N; McNamara, A;
Child, S; Verburgt, L. and Pare, P. (1996). Human airway narrowing measured
using high resolution computed tomography. Am. J. Respir. Crit. Care. Med. 154,
1557 1562.
-
Orsida, B.E; Ward, C; Li, X; Bish, R;
Wilson, J.W; Thien, F, and Walters, E.H. (2001). Effect of a long acting β2 agonist over three months on airway wall vascular remodeling in
asthma. Am. J. Respir. Crit. Care. Med. 164, 117 121.
-
Orsida, B; Li, X; Hickey, B; Thien, F;
Wilson, J, and Walters, E. (1999). Vascularity in asthmatic airways: relation
to inhaled steroid dose. Thorax. 54, 289 295.
-
Overbeek, S.E; Kerstjens, H.A; Bogaard,
J.M; Mulder, P.G, and Postma, D.S. (1996). Is delayed introduction of
inhaled corticosteroids harmful in patients with obstructive airways disease
(asthma
and COPD)? The Dutch Chronic Nonspecific Lung Disease (CNSLD) Study Groups. Chest.
110, 35 41.
-
Paganin, F; Seneterre, E; Chanez, P;
Daures, J.P.; Bruel, J.M; Michel, F.B. and Bousquet, J. (1996). Computed
tomography of the lungs in asthma: influence of disease severity and etiology. Am.
J. Respir. Crit. Care. Med. 153, 110 114.
-
Panettieri, R.A. Jr. (2003). Airway
smooth muscle: immunomodulatory cells that modulate airway remodeling? Respir.
Physiol. Neurobiol. 137, 277 293.
-
Payne, D.N; Rogers, A.V; Adelroth, E;
Bandi, V; Guntupali, K.K; Bush, A. and Jeffery, P.K. (2003). Early thickening
of the reticular basement membrane in children with difficult asthma. Am. J.
Respir. Crit. Care. Med. 167, 78 82.
-
Peat, J; Woolcock, A, and Cullen, K. (
1987). Rate of decline of lung function in subjects with asthma. Eur. J.
Respir. Dis. 70, 171 179.
-
-
Robinson, D.S.,
Hamid, Q., Ying, S., Tsicopoulos, A., Barkans, J and Bentley, A.M. (1992).
Predominant Th2 like bronchoalveolar T
lymphocyte population in atopic asthma. N. Engl. J. Med. 326, 298
304.
-
Roche, W; Williams, J; Beasley, R. and
Holgate, S. (1989). Subepithelial fibrosis in the bronchi of asthmatics. Lancet.
1, 520 524.
-
Rolla, G; Scappaticci, E; Baldi, E, and
Bucca, C. (1986). Methacholine inhalation challenge after rapid saline infusion
in healthy subjects. Respiration. 50, 18 22.
-
Saetta, M., Di Stefano, A., Rosina, C.,
Thience, G. and Fabbri, L. (1991). Quantitative structural analysis of
peripheral airways and arteries in sudden fatal asthma. Am. Rev. Respir.
Dis. 143, 138 143.
-
Saetta, M; Maestrelli, P; Turato, G;
Mapp, C; Milani, G; Pivirotto, F. (1995). Airway wall remodeling after
cessation of exposure to isocyanates in sensitized asthmatic subjects. Am.
J. Respir. Crit. Care. Med. 151, 489 494.
-
Sagara, H; Okada,T; Okumura, K; Ogawa,
H; Ra, C; Fukuda, T, and Nakao, A. (2002). Activation of TGF-b/Smad2 signaling is associated with airway remodeling in asthma. J.
Allergy. Clin. Immunol. 110, 249 254.
-
Salvato, G, (2001). Quantitative and
morphological analysis of the vascular bed in bronchial biopsy specimens from
asthmatic and non asthmatic subjects. Thorax, 56, 902 906.
-
Salvato, G. (2001). Quantitative and
morphological analysis of the vascular bed in bronchial biopsy specimens from
asthmatic and non asthmatic subjects. Thorax. 56, 902 906.
-
Schmidt, M; Sun, G; Stacey, M.A; Mori,
L, and Mattoli, S. (2003). Identification of circulating fibrocytes as
precursors of bronchial myofibroblasts in asthma. J. Immunol. 171,
380 389.
-
Schwarze, J, and Gelfand, E.W. (2002).
Respiratory viral infections as promoters of allergic sensitization and asthma
in animal models. Eur. Respir. J. 19, 341 349.
-
Selroos, O; Pietinalho, A; Lofroos, A.B,
and Riska, H. (1995) Effect of early vs. late intervention with inhaled
corticosteroids in asthma. Chest. 108, 1228 1234. .
-
Sigurs, N; Bjarnason, R; Sigurbergsson,
F, and Kjellman, B. (2000). Respiratory syncytial virus bronchiolitis in
infancy is an important risk factor for asthma and allergy at age 7. Am. J.
Respir. Crit. Care. Med. 161, 1501 1507.
-
Tang, W; Geba, G.P; Zheng, T; Ray, P;
Homer, R; Kuhn, C. (1996). Targeted expression of IL-11 in the murine airway
causes airways obstruction, bronchial remodeling and lymphocytic inflammation. J.
Clin. Invest. 98, 2845 2853.
-
Thurston, G; Rudge, J.S; Loffe, E; Zhou,
H; Ross, L; Croll, S.D. (2000). Angiopoietin-1 protects the adult vasculature
against plasma leakage. Nat. Med. 6, 460 463.
-
Trigg, C; Manolitsas, N; Wang, J;
Calderon, M; McAulay, A; Jordan, S. et al. (1994). Placebo controlled
immunopathologic study of four months of inhaled corticosteroids in asthma. Am.
J. Respir. Crit. Care. Med. 150, 17 22.
-
Ulrik, C; Backer, V, and Dirksen, A.
(1992). A 10 year follow up of 180 adults with bronchial asthma: factors
important for the decline in lung function. Thorax. 47, 14 18.
-
Vignalo, A; Chanez, P; Chiappara, G;
Merendino, A; Pace, E; Rizzo, A. et al. (1997).Transforming growth factor-b expression in mucosal biopsies in asthma and chronic bronchitis. Am.
J. Respir. Crit. Care. Med. 156, 591 599.
-
Vignalo, A; Chanez, P; Chiappara, G;
Merendino, A; Zinnanti, E; Bousquet, J. et al. (1996). Release of TGF b
and fibronectin by alveolar macrophages in airway diseases. Clin.
Exp. Immunol. 106, 114 119.
-
Vrugt, B; Wilson, S; Bron, A; Holgate,
S.T; Djukanovic, R, and Aalbers, R. (2000). Bronchial angiogenesis in severe
glucocorticoid dependent asthma. Eur. Respir. J. 15, 1014 1021.
-
Wang, R.D; Tai, H; Xie, C; Wang, X;
Wright, J.L. and Churg, A. (2003). Cigarette smoke produces airway wall
remodeling in rat tracheal explants. Am. J. Respir. Cri. Care. 168, 1232
1236.
-
Ward, C; Johns, D.P; Bish, R; Pais, M;
Reid, D.W; Ingram, C. (2001). Reduced airway distensibility, fixed airflow
limitation, and airway wall remodeling in asthma. Am. J. Respir. Crit. Care.
Med. 164, 1718 1721.
-
Ward, C; Pais, M; Bish, R; Reid, D;
Feltis, B; Johns, D, and Walters, E.H. (2002). Airway inflammation, basement
membrane thickening and bronchial hyperresponsiveness in asthma. Thorax. 57,
309 316.
-
Wenzel, S.E; Schwartz, L.B; Langmack,
E.L; Halliday, J.L; Trudeau, J.B; Gibbs, R.L. and Chu, H.W. (1999). Evidence
that severe asthma can be divided pathologically into two inflammatory subtypes
with distinct physiologic and clinical characteristics. Am. J. Respir. Crit.
Care. Med. 160, 1001 1008.
-
Wenzel, S.F., Szefler, S.J., Leung, D.Y., Sloan, S.I.,
Rex, M.D. and Martin, R.J. (1997). Bronchoscopic evaluation of severe asthma.
Persistent inflammation associated with high dose glucocorticoids. Am. J.
Respir. Crit. Care. 156, 737 743.
-
Wills-Karp, M; Luyimbazi, J; Xu, X;
Schofield, B; Neben, T.Y; Karp, C.L, and Donaldson, D.D. (1998).
Interleukin-13: central mediator of allergic asthma. Science. 282, 2258
2260.
-
Wilson, J. and Li, X. (1997). The
measurement of reticular basement membrane and submucosal collagen in the
asthmatic airway. Clin. Exp. Allergy. 27, 363 371.
-
Woodruff, P.G; Dolganov, G.M; Ferrando,
R.E; Donnelly, S; Hays, S.R; Solberg, O. (2004). Hyperplasia of smooth muscle
in mild to moderate asthma without changes in cell size or gene expression. Am.
J. Respir. Crit. Care. Med. 169, 1001 1006.
-
Zhu, Z; Homer, R.J; Wang, Z; Chen, Q;
Geba, G.P; Wang, J. (1999). Pulmonary expression of interleukin-13 causes
inflammation, mucus hypersecretion, subepithelial fibrosis, physiologic abnormalities
and eotaxin production. J. Clin. Invest. 103, 779 788.
© Physiological Society of Nigeria 2005
|