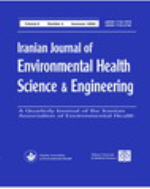
|
Iranian Journal of Environmental Health, Science and Engineering
Iranian Association of Environmental Health (IAEH)
ISSN: 1735-1979
Vol. 4, Num. 2, 2007, pp. 77-84
|
Untitled Document
Iranian Journal of Environmental Health Science & Engineering,Vol.
4, No. 2, 2007, pp. 77-84
PERFORMANCE EVALUATION OF AN ANAEROBIC BAFFLED REACTOR TREATING
WHEAT FLOUR STARCH INDUSTRY WASTEWATER
1H. Movahedyan, *2A. Assadi, 1A. Parvaresh
1Department of Environmental Health Engineering, Isfahan University of Medical Sciences,
Isfahan, Iran
2Department of Environmental Health Engineering, Zanjan University of Medical Sciences, Zanjan, Iran
*Corresponding author-Email: assadi@zums.ac.ir Tel: +98 241 7281301,
Fax: +98 241 7281317
Received 19 December 2006; revised 15 January 2007; accepted 30 March 2007
Code Number: se07012
ABSTRACT
Feasibility of the anaerobic baffled reactor process was investigated for
the treatment of wheat flour starch wastewater. After removal of suspended
solids by simple gravity settling, starch wastewater was
used as a feed. Start-up of a reactor (with a volume of 13.5 L and five compartments)
with diluted feed
of approximately 4500 mg/L chemical oxygen demand was accomplished in about
9 weeks using seed
sludge from anaerobic digester of municipal wastewater treatment plant. The
reactor with hydraulic
retention time of 72h at 35°C and initial organic loading rate of 1.2 kgCOD/m3.d
showed 61% COD removal efficiency. The best performance of reactor was observed
with an organic loading rate of 2.5
kgCOD/m3.d or hydraulic retention time of 2.45 d and the COD conversion of
67% was achieved. The
system also showed very high solids retention with effluent suspended solids
concentration of about 50
mg/L for most organic and hydraulic loadings studied. Based on these observations,
the ABR process
has potential to treat food industrial wastewater as a pretreatment and is
applicable for extreme
environmental conditions.
Key words: Anaerobic baffled reactor, starch wastewater, COD removal, organic loading
INTRODUCTION
During the last 30 years, there has been
an increasing demand for more efficient systems
for the treatment of wastewater due to
increasingly stringent discharge standards now widely
adopted by various national and international
agencies (Akunna and Clark, 2000). The treatment of
both domestic and industrial wastewater is usually carried out using biological methods due to
their lower costs compared to chemical methods (Langenhoff et al., 2000). Great advances have been made over the last 20 years in
anaerobic reactor design and in understanding the
complex processes that occur in anaerobic
digestion (Langenhoff and Stucky, 2000). The
successful application of anaerobic systems to the
treatment of industrial wastewater is critically dependent
on the development and use of high rate anaerobic bioreactors. These reactors achieve a
high reaction rate per unit reactor volumes in terms
of kgCOD/m3.d by retaining the biomass Solid Retention Time, (SRT) in the reactor
independently of the incoming wastewater Hydraulic
Retention Time, (HRT), (Barber and Stucky, 1999).
Such separation allows the slow growing anaerobic bacteria remain within the reactor independent
of the wastewater flow. This allows higher
volumetric loads and produces significantly enhanced
removal efficiencies (Akunna and Clark, 2000). In
contrast to domestic wastewater, industrial effluents
pose many problems for treatment and such
effluents are subject to daily and sometimes
seasonal fluctuations with respect to both their flow
and strength (Nachaiyasit and Stucky, 1997a). The characteristics of food-processing wastes
show high variation in Biological Oxygen Demand (BOD), Total Suspended Solids (TSS) and
flow rate. The wastes may be highly alkaline or
highly acidic. Mineral materials (N and P) may be
absent or may be present in excess of the ratio
necessary to promote good environmental conditions
for biological treatment (Nemerow and Dusgopta, 1991). Wheat starch is an important agro-based product found in many parts of the world.
The starch extraction process includes
preprocessing of wheat flour, starch extraction, separation
and drying (Rausch, 2002). It produces large
volume of wastewater up to 20-60 m3/T of
starch produced. Water pollution problems related to
the starch industry are serious. The wastewater is highly organic and acidic by nature with COD
up to 25000 mg/L and pH between 3.8-5.2 (Annachhatre and Amatya, 2000). High
rate anaerobic processes offer an attractive
alternative for treatment of starch industry
wastewater (Lettinga, 1996). The most common high
rate anaerobic reactor in the world today is the
Upflow Anaerobic Sludge Blanket (UASB), with many existing full-scale reactors for the treatment
of wastewater from the food and beverages
industries (Austermann-Houn et al., 1999). The success
of the UASB depends on the formation of active and settlable granules. These granules are involved
in aggregations of anaerobic bacteria and self-immobilized into compact forms (Yan and
Tay, 1997). Due to the relatively low densities of
the UASB granules, the loss of biomass with
effluent poses problems, especially during increased
loading rates (Angenent et al., 2002). One of
the many types of high rate reactors presently attracting growing interests is the
Anaerobic Baffled Reactor (ABR) developed by
McCarty and coworker (1981) at Stanford University.
The ABR has been described as a series of UASBs which does not require granulation for its
operation. Therefore, it has lower start-up period than
the other high rate reactors. It includes a series
of vertical baffles to force the wastewater to
flow under and over them, and therefore the
wastewater comes into contact with a large active
biomass (Nachaiyasit and Stucky, 1997a). This
reactor system has been reported to have many advantages over other systems. The
most significant advantage of the ABR is its ability
to separate acidogenesis and methanogensis longitudinally down the reactor, allowing the
reactor to behave as a two-phase system without
the associated control problems and high costs
(Barber and Stucky, 1999). It is simple in design and
requires no gas separation systems. Moreover, the hydrodynamic pattern reduces bacterial washout and enables it to retain biomass without the use
of any fixed media (Grove et al., 1999; Vossoughi et al., 2003). The separation of two phases
causes an increase in protection against toxic material
and higher resistance to changes in environmental parameters such as pH, temperature and
organic loading (Barber and Stucky, 2000; Uyanik et al., 2002). Among high rate reactors, ABR
was recommended by several researchers as a promising system for industrial and also
domestic wastewater treatment (Nachaiyasit and
Stucky, 1997b; Barber and Stucky, 1999; Wang et
al., 2004). The objective of this study was
evaluation of the performance of the ABR during
various hydraulic and loading conditions.
MATERIALS AND METHODS
Experimental set-up
The laboratory scale ABR was constructed
from 6mm thick transparent Plexiglas, with
external dimensions of 53cm long, 16cm width and a
depth of 30cm, with the working volume 13.5L. Fig.
1 shows a schematic diagram of the reactor. The reactor was divided into five equal 2.7
L compartments by vertical baffles, each compartment having down comer and riser
regions created by a further vertical baffle. The width
of up comer was 2.6 times of the width of down
comer. The lower parts of the down comer baffles
were angled at 45º in order to direct the flow
evenly through the up comer. This produced
effective mixing and contact between the wastewater
and anaerobic sludge at the base of each riser.
Each compartment was equipped with sampling ports
that allowed biological solids and liquid samples to
be withdrawn. The operating temperature was maintained constant at 35±0.5ºC by putting
the reactor in a water bath equipped with a
temperature regulator. The influent feed was pumped using
a variable speed peristaltic pump (Master flux
L/S). The outlet was connected to a glass U-tube
for level control and to trap solids. The produced
gas was collected via portholes in the top of the
reactor and daily volume was determined using the
gas-water displacement technique.
Wastewater characteristics
The combined starch wastewater from a
wheat starch factory in the central province of Iran (Ardineh starch CO, Isfahan) was used as
feed. Wheat starch industry combined wastewater
has low pH, high suspended solids and high COD.
The supernatant of the combined wastewater after
the simple gravity settling used in the investigation,
had lower TSS, as approximately 90% of the solids were removed. Typical characteristics of
the wheat starch wastewater are given in Table 1.
The supernatant wastewater was diluted to
achieve the COD concentration required for each
loading rate with tap water. In order to pH and
alkalinity adjustment, the supernatant was neutralized
by NaOH and NaHCO3. A COD:N:P ratio of
300:5:1 was kept during operation using
NH4Cl and K2HPO4. The micro-nutrient deficiency
was added occasionally to correct growth
conditions according to Angenent et al., (2002).
Seed sludge
The reactor was seeded with anaerobic
digested sewage sludge taken from an anaerobic
digester an Isfahan south municipal sewage treatment works. It was first sieved (<2mm) to remove
any debris and large particles and was then
introduced into five compartments of reactor. Each compartment was filled with 50% sludge
which had a suspended solids composition of 30980
mg TSS/L and 20880 mg Volatile Suspended Solids (VSS) per liter giving a total of 140.94 g VSS
in the reactor. This value (10.44 g VSS/L) is in accordance with the initial VSS value used in
other studies with ABR (Barber and Stucky, 1999).
The remaining parts of each compartment were
filled with tap water. After seeding the reactor, the
lids were sealed. The reactor was then allowed to stabilize for 24h without further modification
before starting the experiments.
Analysis
Monitoring included the analysis of samples
from influent and each compartment of the ABR
system for COD, TSS, VSS, alkalinity and pH. All
samples were analyzed according to the standard
methods for examination of water and wastewater (APHA,1995). pH and temperature
were monitored daily.
RESULTS
Reactor start-up
In order to reactor start-up, the batch operation of ABR was
started using an initial sludge concentration of 10440 mg VSS/L. The system
was run on batch for 2 weeks. During this time the content of the reactor was
recycled
for homogeneity. After these weeks the ABR was run continuously and observation
were made for 42 days with an initial organic loading rate of
1.2 kgCOD/m3.d with influent COD in the range
of 4225-5975 mg/L. When there was no more fluctuation in COD in each compartment, then
the Organic Loading Rate (OLR) was increased up to 1.5
kgCOD/m3.d for one week and then OLR were increased gradually up to 10
kgCOD/m3.d for 40 days. At the end of this period, the
biogas production rate increased (data not shown).
COD removal
OLR and HRT employed during the
reactor operation period are shown in Fig.
2. As can
be seen, there were an inversely relationship
between OLR and HRT. Fig.
3 shows the effects of increase of influent COD concentration on
COD removal rate under steady-state conditions. It
also indicates the reactor effectiveness as percent
COD removal. According to Fig.
2 and Fig.
3, the
COD conversion during the start-up period was
obtained up to 61%, with HRT of 3 days (OLR of 1.5
kgCOD/m3.d). After each increase in OLR
the COD concentration in effluent increased.
When the OLR was increased to 2.5, 5 and 10
kgCOD/m3.d at HRT in the range of 1.43-2.45 d,
the efficiency of COD removal was achieved as 67%, 55% and 40%, respectively. Fig.
4 shows the
COD removal efficiency based on organic loading
rates. The best performance of reactor was observed
with an OLR of 2.5 kgCOD/m3.d (HRT of 2.45
d) and the COD conversion of 67% was achieved
(Fig.
4). The variations of COD in the reactor are illustrated in Fig.
5.
Alkalinity and pH
As shown in Fig.
6, the feed alkalinity after
pH adjustment was approximately 1000-3000 mg/L, while the effluent alkalinity was always in the
range of 2000-5000 mg/L. The results of pH
variations along with the reactor showed that the pH decreases in compartments 1 and 2 upto
pH<6.5 during the reactor operation. However,
although the pH in compartments 3-5 returned to
near neutrality, compartment 1 stayed at
approximately pH=6.4 for the rest of the experiment. This
is clearly shown in Fig.
7.
Sludge characterization
The average TSS and VSS concentration
and VSS/TSS ratio in the reactor at different
times are presented in Fig.
8. At the time of reactor
start-up, VSS/TSS was 0.67. The VSS/TSS ratio increased upto 0.77 by day 42 and later it
slightly decreased till the end of operation. Sludge
washout was negligible in the experiments since the
effluent suspended solids concentrations were in the
range of 14-272 mg TSS/L, which indicates that the
ABR was stable to high shock loads. Details of
reactor operation data are shown in Table 2.
DISCUSSION
The reactor operation lasted for three
months. Because of increment different influent COD
at each period from wide range of 4225 to 14300 mg/L, the COD removal rate (in term of
mg/L.d) increased in accordance with OLR
increasing. However, in spite of incremental trend in
COD removal rate, when the reactor was fed with an organic loading rate of 5
kgCOD/m3.d after 69 days, the treatment removal efficiency
decreased. This is obviously illustrated in Fig.
3. It is
evident from data that the COD removal rate did not
gratify in comparison with lower organic loading
rates. Since in the present study at higher OLR,
the influent COD concentration and volume of flow rate increased significantly and affected
the quantity of COD removal rate. It also led to
high COD concentration in the effluent. The
relatively poor performance of ABR at higher
organic loading rates can be attributed to the presence
of high substrate gradient. In this study, the
best reactor performance was observed with an OLR of 2.5
kgCOD/m3.d and 67% COD removal efficiency was obtained (Fig.
4). The highest
OLR tested (10 kgCOD/m3.d) resulted in only
40% decrease in the COD concentration and the remaining high COD in the effluent. This high
OLR and high flow rate probably caused channeling through the biomass bed, resulting in
poor substrate-biomass contact and minimal
degradation of the incoming COD. This provides
further support to the earlier supposition that under
plug-flow conditions, incoming substrate remains in
the reactor for one retention time allowing
maximum time for conversion. However, the high
substrate concentration resulting from lack of dispersion
may inhibit bacterial activity (Sallis and Uyanik, 2003).
These results support the findings of the
other studies. Kalyuzhnyi et al., (1998) worked
with UASB reactor for chip-processing industry wastewater. The organic loading rate achieved
in the lab scale reactor had approximately 14 gCOD/L.d with treatment efficiencies higher than
75% and 63% on the basis of centrifuged and total
COD of the effluent. Grover et al., (1999) have
shown that a maximum COD reduction of about 60% at an organic loading rate of 5
kgCOD/m3.d at hydraulic retention time of 2d and 35ºC
was recorded with anaerobic baffled reactor
treating pulp and paper liquors. In other study to
treat tapioca starch industry wastewater with UASB process, COD removal upto 95% has been obtained at an OLR of 16
kgCOD/m3.d (Annachhatre and Amayta, 2000). A
comparison of the results achieved with those reported for
other anaerobic treatment systems and for similar wastewaters illustrate that our results
are satisfactory, though an actual comparison
between various data sets can be based only on
experiments where the same wastewater and reactors
of comparable size with the same operation temperature are used.
The variation in the COD profile in ABR was shown in Fig.
5. As it can
be seen, most of COD was removed in compartment 1 (35%), increasing the initial OLR enhanced
the biological oxidation upto a certain point at
which OLR started to inhibit the degradation rate. Because in strong wastewater containing
high organic load, significant amounts of fatty acids
can develop from partial degradation of substrate
and these can inhibit the methanogenic population
in the reactor (Uyanik et al., 2002).
According to Fig.
6, the effluent alkalinity was
always more than the feed alkalinity. The most likely explanation for this observation is
the formation of HCO3- due to the reaction of
OH- with CO2 produced during anaerobic
degradation (Annachhatre and Amayta, 2000). The pH
levels in the reactor followed a similar pattern
throughout the experiment, dropping rapidly to pH less
than 6.4 at reactor start-up and at higher OLR (Fig.
7). The pH decrease in compartments 1 and 2 can be attributed to the fact that high concentration
of volatile fatty acids (VFAs) were present in
these compartments, while in later compartments
due to conversion and stabilization of
intermediate products i.e. VFAs to methane and activity
of methanogenic bacteria the pH value increased to neutral range (Uyanik et al., 2002). Very little washout of the reactor solids occurred in
the reactor operation (Table 2), which show that
the reactor was stable to severe shocks loads. This
is consistent with the published literature which indicates that ABR process is stable to
large transient shock loads (Nachaiyasit and
Stucky, 1997b). Determination of VSS/TSS ratio
gives correlation to the biomass growth and its
quality. As it can be seen in Fig.
8, at the start-up
of reactor, VSS/TSS ratio was 0.67 and increased
to 0.77 by day 42 at OLR of 1.2
kgCOD/m3.d. After this period, it gradually decreased over the
entire course of experiment to 0.73 by day 79 at OLR
of 10 kgCOD/m3.d. This provides further support
to the earlier hypothesis that biomass growth rate
is limited in high organic loading rate (Metcalf
& Eddy, 2003). As a final conclusion, it could
be concluded that this type of reactor
configuration has potential in treating food industrial
wastewater that vary in both flow and concentration.
Also because of equalization characterization ABR process is a good system as pretreatment for
other anaerobic or aerobic wastewater treatment processes.
ACKNOWLEDGEMENTS
The authors are grateful for the financial support
of the research vice chancellor of Isfahan University of Medical Sciences; Project
# 83389. Also, special thanks go to valuable cooperation
of Ardineh Starch CO., for providing feed samples.
REFERENCES
- Akunna, J. C., Clark, M., (2000). Performance of
granular-bed anaerobic baffled reactor (GRABBR) treating
whisky distillery wastewater. Bioresource. Technol., 74: 257-261.
- Angenent, L. T., Abel, S. J., Sung, S., (2002). Effect of
an organic shock load on the stability of an anaerobic
migrating blanket reactor. J. Environ. Eng., 128 (12): 1109-1120.
- Annachhatre, A. P., Amatya, P. L., (2000). UASB
treatment of tapioca starch wastewater. J. Environ. Eng. 126 (12):
1149-1152.
- APHA, AWWA, WEF., (1995). Standard Methods for
the Examination of Water and Wastewater. 19 th Ed., Washington.DC.
- Austermann-Houn, U., Meyer, H., Seyfried, C.
F., Rosenwinkel, K. H., (1999). Full scale experience
with anaerobic/aerobic treatment plants in the food and
beverage industry. Wat. Sci. Technol., 40 (1): 305-312.
- Barber, W. P., Stuckey, D. C., (1999). The use of
anaerobic baffled reactor (ABR) for wastewater treatment: a
review. Water. Res., 33 (7): 1559-1578.
- Barber, W. P., Stuckey, D. C., (2000). Metal
bioavailability and trivalent chromium removal in ABR. J. Environ.
Eng., 126 (7): 649-656.
- Grover, R., Marwaha, S. S., Kennedy, J. F., (1999).
Studies on the use of an anaerobic baffled reactor for the
continuous anaerobic digestion of pulp and paper mill black
liquors. Process Biochem., 39: 653-657.
- Kalyuzhnyi, S., Santos, L., Martinez, J. R., (1998).
Anaerobic Treatment of Raw and Preclarified
Potato-Maize Wastewater in a UASB Reactor. Bioresource.
Technol., 66: 195-199.
- Langenhoff, A. M., Intrachandra, N., Stucky, D. C.,
(2000). Treatment of dilute soluble and colloidal wastewater using an anaerobic
baffled reactor: influence of hydraulic
- retention time. Water. Res., 34 (4): 1307-1317.
- Langenhoff, A. M., Stuckey, D. C., (2000). Treatment
of dilute wastewater using an anaerobic baffled reactor:
effect of Low temperature. Water. Res., 34 (15): 3867-3875.
- Lettinga, G., (1996). Sustainable integrated
biological wastewater treatment. Water. Sci. Technol., 33 (3): 85-98.
- Metcalf and Eddy., (2003). Wastewater engineering,
treatment and reuse., 4th Ed. McGraw Hill Inc, 984-988.
- Nachaiyasit, S., Stucky, D. C., (1997a). The effect of
shock loads on the performance of an anaerobic baffled
reactor (ABR), 1. Step change in feed concentration at
constant retention time. Water. Res., 31 (11): 2737-2746.
- Nachaiyasit, S., Stucky, D. C., (1997b). The effect of
shock loads on the performance of an anaerobic baffled
reactor (ABR), 2. Step and transient hydraulic shocks at
constant feed strength. Water. Res., 31 (11): 2747-2754.
- Nemerow, N. L., Dasgupta, A., (1991). Industrial
and hazardous waste treatment., VNR Inc., 421-422.
- Rausch, K. D., (2002). Front end to backpipe: membrane technology in the
starch processing industry. starch/stärke., 54: 273-284.
- Sallis, P. J., Uyanik, S., (2003). Granule development in
a spilt-feed anaerobic baffled reactor. Bioresource.
Technol., 89: 255-265.
- Uyanik, S., Sallis, P. J., Anderson, G. K., (2002). The
effect of polymer addition on granulation in an anaerobic
baffled reactor (ABR). Part. I: Process performance. Water.
Res., 36: 933-943.
- Vossoughi, M., Shakeri, M., Alemzadeh, I.,
(2003). Performance of an anaerobic baffled reactor
treating synthetic wastewater influenced by decreasing
COD/SO4 ratio. Chem. Eng. Processing., 42: 811-816.
- Wang, J., Huang, Y., Zhao, X., (2004). Performance
and characteristics of an anaerobic baffled reactor.
Bioresource. Technol., 93: 205-208.
- Yan, Y. G., Tay, J. H., (1997). Characterization of
the granulation process during UASB start-up. Water.
Res., 31 (7): 1537-1580.
© 2007 Tehran University of Medical Sciences Publications
The following images related to this document are available:
Photo images
[se07012f5.jpg]
[se07012f2.jpg]
[se07012f7.jpg]
[se07012t2.jpg]
[se07012f8.jpg]
[se07012f1.jpg]
[se07012t1.jpg]
[se07012f4.jpg]
[se07012f6.jpg]
[se07012f3.jpg]
|