Untitled Document
Iranian Journal of Environmental Health Science & Engineering,Vol.
4, No. 2, 2007, pp. 85-92
REMOVAL OF ARSENIC FROM AN AQUEOUS SOLUTION BY PRETREATED
WASTE TEA FUNGAL BIOMASS
1S. Mamisahebei , *1Gh. R. Jahed Khaniki, 2A. Torabian, 1S. Nasseri, 1K. Naddafi
1Department of Environmental Health Engineering, School of Public Health, Medical Sciences/University of Tehran,
Tehran, Iran
2Department of Civil Environmental Engineering, Faculty of the Environment/University
of Tehran, Tehran, Iran
*Corresponding author-Email: ghjahed@tums.ac.ir Tel: +98 21 8895 4914,
Fax: +98 21 8895 0188
Received 28 December 2006; revised 30 February 2007; accepted 25 March 2007
Code Number: se07013
ABSTRACT
Arsenic contamination in water poses a serious threat on human health. The
tea fungus known as Kombucha is a waste produced during black tea fermentation.
The objective of this study was to examine
the main aspect of a possible strategy for the removal of arsenates employing
tea fungal biomass. The
pretreatment of biomass with FeCl3 was found to improve the biosorption efficiency.
Arsenics uptake was
found to be rapid for all concentrations and reached to 79% of equilibrium
capacity of biosorption in 20
min and reached equilibrium in 90 min. The pseudo second-order and first-order
models described the
biosorption kinetics of As (V) with good correlation coefficient (R2>0.93)
and better than the other equations. The data obtained from the experiment
of biosorption isotherm were analyzed using the
Freundlich and Langmuir isotherm models. The equation described the isotherm
of As (V) biosorption with
relatively high correlation coefficient (R2>0.93). According to the Langmuir
model, the maximum uptake capacities (qm) of tea fungal biomass for As (V)
were obtained 3.98×10-3 mmol/gr. The effect of Na+, K+,
Mg+2 and Ca+2 on equilibrium capacities of As was not significant. The variation
of sorption efficiency with
pH showed that optimum biosorption takes place in the pH ranges of 6 to 8.
Promising results were
obtained in laboratory experiments and effective As (V) removals were observed.
Key words: Waste tea, fungal biomass, biosorption, arsenates isotherm, aqueous solution
INTRODUCTION
Arsenic exists in natural waters both in
inorganic and organic forms. The inorganic form of
arsenic is more toxic compared to its organic
form. Inorganic arsenic is the predominant form in contaminated groundwater. Arsenic exists
in two,oxidation states: arsenite, As (III) and
arsenate, As (V) (Pokhrel and Viraraghavan, 2006). It
is introduced in the aqueous system through geochemical reactions industrial waste
discharge or even agricultural use of arsenical
pesticides (Loukidou et al., 2003). The presence of
arsenic in groundwater has been a major public
health concern in many countries such as
Bangladesh, India, China, Kurdistan of Iran, Argentina,
Canada and USA (ATSDR, 2002 and WHO, 2001). The problem of arsenic contamination in groundwater poses is a serious threat in these areas
because groundwater is the main source of drinking
water (Pal et al., 2002). Long-term exposures to
arsenic levels can result in permanent and severe
damage to human health. Arsenic toxicity causes
skin lesions, damage mucous membranes, digestive, respiratory, circulatory and nervous
system (Murugesan et al., 2006). According to
the European Commission as well as to the US Environmental Protection Agency, the
acceptable value of As in drinking water is limited to10
µg/L from an earlier value of 50 µg/L (Loukidou et al., 2003). Removal of arsenic from
contaminated water to satisfy the drinking water standard
has been a challenge for water authorities. Iron
oxides have been reported to be effective for metal
ion removal. Iron oxide-coated sand was used in
many studies for arsenic removal (Pokhrel and Viraraghavan, 2006; Joshi and Chaudhuri,
1996). Huang and Vane (1989) treated activated
carbon by various cationic metal salt solutions, such
as Ba(ClO4)2,
Cu(ClO4)2, FeSO4,
FeCl2, Fe(ClO4)2 , Fe(ClO4)2,
Fe(ClO4)3 and FeCl3 .They found that arsenate removal by activated carbon pretreated
with the ferrous salt was the best among other solutions (almost ten times greater than
untreated activated carbon). In Bangladesh,
groundwater rate of As (V)/As (III) is variable from 1.1 to
10, but the usual rate of this index is 1.66 to 2
(Smedley and Kinniburgh, 2002). The research for
highly biomass sorption is expected to improve the potential for the introduction of new
materials, which are likely to be competitive and
cost-efficient for the sorption of metals. The objective of
this study was to examine a possible strategy for
the removal of arsenates, employing tea fungal biomass.
MATERIALS AND METHODS
Preparation of tea fungus
Tea fungus (a simbiont of two yeasts Viz.,
Pichia sp. NRRL Y-4810 and Zygosaccharomyces sp. NRRL Y-4882 and a bacterium Acetobacter
sp. NRRL B-2357) was obtained from perfumery of Tehran city. The fungus was grown in tea
medium. To 1 L of water, two tea spoons (10.5 g) of
tea powder was mixed and boiled for 5 min. Then 100 g of sucrose was added. The tea extract
was filtered and used as a medium. It was
incubated at 28±2°C statically for ten days. New fungal
mat was produced over the mother culture
(Murugesan et al., 2006).
Biosorbent preparation
The tea fungal mat was washed with an
adequate amount of deionized water until free
media components. The washed fungal mat was dried at
75°C for 24 h and the dried biomass was
ground in a laboratory blender. Then a portion of the
dried and ground mat was immersed in
FeCl3 solution (15 mg/L) for 60 min. These tow modified
fungal mats were then tested for efficacy to remove
As (V) from an aqueous solution.
Chemicals
Synthetic solutions were prepared by the use
of deionized water and salts including NaCl, KCl, MgCl2 and
CaCl2 (Merck supplied). Initial pH of the solutions was adjusted with electrical pH
meter (CAMLAB Lts, Model CG 842) to the desired values by using 0.1 to 1 mol/L HCl and 0.1 to
1 mol/L NaOH.
Equilibrium experiments
The optimum speed of agitation was adjusted
about (50-250 rpm). The solution volume was 100 mL
in all batch equilibrium experiments. The sample
was agitated in a rotary shaker at 160 rpm. All experiments were conducted at room
temperature (22±4°C). The initial pH of solutions was
adjusted to desired values and then the final pH
was measured.
Biosorption isotherms
Biosorption isotherm experiments were
conducted in a single-component system. The initial As
(V) concentration was 1 mg/L and biosorbent doses varied from 0.1 to 1 g. Also, the initial pH
of solutions was adjusted to 6.
Effect of pH on
biosorption
The effect of pH on equilibrium capacities of
As (V) biosorption was studied in a
single-component system. The initial As (V) concentration was
1 mg/L and the pH of solutions had some variations from 2 to 9. After pH adjustment, 100 mg of
tea fungus biomass was added to experiment vessels.
Effect of background
ions
Ionic species such as
Ca2+, Mg2+, K+ and
Na+ are usually present in drinking water and
groundwater contaminated with arsenic (Smedley and Kinniburgh, 2002). These ions may interfere in
the uptake of arsenic by adsorbent through
competitive binding or complexion.
Metal analysis
The biomass was removed by filtration
through 0.45 mm membrane filters (Mixed cellulose esters).Then the filtrates were analyzed
for residual As (V) concentration by ICP model AMS 100 "l-speeder".
Kinetic modeling
Kinetic of As (V) biosorption was modeled by
the pseudo first-order (Langergren), pseudo second-order, saturation (mixed-order) and
second-order rate equations, presented as equations 1 to 4,
respectively:


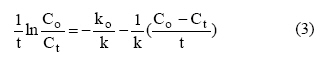

Where qe and
qt are the amount of metal ion sorbed (mmol/g) at equilibrium at any time,
respectively; K1 is the pseudo first-order rate constant
of adsorption (1/min); K2 is the pseudo
second-order rate constant of adsorption (g/mol/min),
Co and Ct are the metal concentrations.
Isotherm modeling
In order to estimate the maximum capacities
of adsorbents, it is necessary to know the quantity
of adsorbed metal as a function of metal concentration in the solution.
Typically two models can be applied
as Freundlich and Langmuir isotherms.
- Freundlich isotherm
The Freundlich equation serves as a tool
for simulating adsorption processes and
equation is given by equations 5 and 6.
q = K. Cn (5)
or log q = log K + n log C
(6)
Where q (mg/g) and C (mg/mL) are
the equilibrium concentrations of solute in solid
and liquid phases, respectively. The Freundlich constants K (mg/g) and n (value between 0
and -1) represent the adsorption capacity and adsorption intensity of the
adsorbent, respectively.
- Langmuir isotherm
The Langmuir equation is based on the
assumption that maximum adsorption corresponds to
a saturated monolayer of salute on the adsorbent
surface, and that the energy of adsorption is constant and there is no transmigration
of adsorbate in the plane of the surface. The Langmuir equation is given by
equations (7).
q=qmaxC/
(C+Kd) (7)
Constants qmax (mg/g) and
Kd (mg/mL) are the Langmuir parameters. The constant
qmax represents the maximum adsorption
capacity and Kd is the dissociation coefficient of
the soluteadsorbent complex, which represents the affinity between the solute and the
adsorbent. The Langmuir isotherm has been widely accepted as a practical method for
integrating experimental data of adsorption
processes. Rearranging equations. (8), a linear
expression can be derived as a function of C:
C/q=Kd/qmax+(1/q max)C (8)
RESULTS
Kinetic study
The kinetic profile of As (V) biosorption by
tea fungus is shown in Fig. 1.
Kinetic parameters of rate equations
for biosorption of As (V) by tea fungus biomass
are shown in Tables 1 and 2.
Fig. 2. (a)-(d) show kinetic modeling of As
(V) biosorption by linear plots of the pseudo first
-order, pseudo second-order, saturation and second
rate equation (Equations 1 to 4).
Isotherm study
Fig. 3. (a)-(b) shows isotherm modeling of As
(V) biosorption by linear plot of the Freunlich
and Langmuir models (Equations 5 to 8).
Effect of pH on biosorption
Fig. 4 shows the effect of pH on equilibrium
uptake capacities of tea fungus biomass for As (V).
Effect of light metal ions on biosorption
The effect of Na+,
K+, Mg2+ and Ca2+ on
equilibrium
capacities of As (V) biosorption by tea fungus
biomass is shown in Fig. 4.
Effect of agitation speed
The influence of agitation speed on adsorption
of As (V) on tea fungal biomass was studied by changing the speed of agitation from 50 to
250 rpm and result are shown in Fig. 6.
DISCUSSION
Kinetic study
According to Fig. 1, As (V) uptake was
relatively fast for all concentrations studied. At the initial
As (V) concentration of 4 mg/L, the system reached to equilibrium within 90 min. In general the
heavy metals uptake reached to 79% equilibrium
capacity of biosorption in 15 min. This rapid kinetic
has significant practical importance as it will
facilitate smaller reactor volumes ensuring efficiency
and economy. Similar rapid metal uptake has been reported for the biosorption of As (V)
using P.chrysogenum where in the system reached
over 60-70% equilibrium uptake capacity in 10 min (Loukidou et al., 2003). The biosorption of As (V) by Kombucha biomass was similar and 67%
of the total uptake occurred in 70 min (Murugesan et al., 2006). In present study, the pseudo
first-order, pseudo second-order and saturation
rate equations described the biosorption kinetic of
As (V) with good correlation coefficient
(R2> 0.93). Kinetic analysis of As (V) biosorption
by pretreated waste tea fungal biomass represented that the pseudo first-order rate equation described
biosorption kinetic better than rest (Murgesan et al., 2006). The rate constant of the pseudo
second-order rate equation for As(V) biosorption
were obtained as 10.38, 1.26 and 1.14 g/mmol/min
at the initial As(V) concentrations of 1, 2 and 4
mg/L, respectively. An increase in initial
concentration of As(V) led to a decrease in the rate
constant value. Therefore there was not a direct relationship between initial concentration of As(V)
and the rate of As(V) biosorption by tea
fungal biomass. In other hand, the biosorption of As
(V) tea fungal biomass was slower in high initial
metal ion concentration. Analysis of equilibrium data
is important for developing an equation that can
be used for design purposes. Several isotherm equations have been used for the
equilibrium modeling of biosorption systems (Sag and Kutsal, 1995). In this study, Langmuir and
Freundlich isotherms have been applied. For each
isotherm the initial As (V) concentrations was kept
constant whereas the biomass concentration were
varied between 0.1-1 mg in each container with
0.1L volume. The Langmuir equation described the isotherm As (V) biosorption by tea fungal
biomass with high correlation coefficient
(R2>0.99) and it was better than the Freundlich model
(R2> 0.95). According to Langmuir equation the
maximum capacity of As (V) biosorption
(qm) was obtained as 3.98×10-3 (mmol/g). The Langmuir parameters of
qm (maximum uptake capacity) is a
suitable measure by comparing different sorbents for
the same sorbate. Although due to the
various experimental conditions employed in
different studies, comparison of their results is difficult.
The effect of pH on As (V) biosorption was studied
in the initial pH range from 2 to 12. The optimum initial pH values for As (V) biosorption
were determined as 6 to 8. The sharpest increase in
As (V) uptake was obtained between pH=3 and 5. Measurement of final pH represented
the simultaneous release of H+ with the uptake
of arsenic ions, because final pH of solutions was
less than the initial pH. Therefore ion
exchange was confirmed to be one of the
biosorption mechanisms. The relative distribution of
dissolved arsenic is influenced by pH and the
redox conditions. Under mildly reducing conditions
and at pH=6 and 9, As (V) exists predominantly as
H2As2SO4- and is likely to form a complex with iron oxide-coated biomass. At higher pH, OH
ions in the solution can compete with the arsenite
ions and so adsorption of As (V) will be reduced (Pokhrel and Viraraghavan, 2006).
Ionic species such as
Ca2+, Mg2+, Na+ and
K+ are usually present in drinking water and
groundwater contaminated with arsenic (Smedley and Kinniburgh, 2002). Batch equilibrium
experiments were conducted to evaluate the influence of
Ca2+, Mg2+, Na+ and
K+ on As (V) removal by pretreated tea fungal biomass. As (V) removal obtained
from experiments with Ci=1 mg/L and several
initial concentrations of Ca2+,
Mg2+, Na+ and K+ are displayed in Fig. 5. Within the initial concentration
range of 50-100 mg/L, K+ ,
Ca2+ and Mg2+ had effect on As (V) removal by about
4-10%. Increasing the initial concentrations of
background ions to 500 mg/L caused decrease on As
removal.
It is evident from Fig. 6 that the removal of
As (V) increased with the increase in agitation
speed from 50 to 150 rpm. This may be explained by
the fact that the increasing agitation rate
decreases the boundary layer resistance to mass transfer
in the bulk and increases the driving force of
As(V) ions. It may be, therefore, assumed that the
film difusion does not dominantly control the
overall adsorption process (Mckay, 1981). It is
further noted that there was no significant increase
in uptake above 150 rpm. The results revealed that
FeCl3 treated and autoclaved fungal mats
are efficient in removing As (III), As (V) and Fe
(II) from groundwater samples, respectively.
FeCl3 treated fungal mat work better because iron
has an affinity towards arsenic in forming
arsenic-iron oxides. Also,some researchers had reported
that iron has more affinity toward arsenic (Hodi et al., 1995; Singh et al., 1998; Welch et al., 2000; and Thirunavukkarasu et al., 2003). Hence
FeCl3 pretreated fungal mat work better than
nontreated mat and we achieved the samed result in this study.
ACKNOWLEDGEMENTS
The authors are most grateful to laboratory
staff of the Department of Environmental Health Engineering, School of Public Health,
Tehran University of Medical Sciences and
Laboratory staff of the Department of Environmental
Civil Engineering, University of Tehran for their collaboration in this research.
REFERENCES